the Creative Commons Attribution 4.0 License.
the Creative Commons Attribution 4.0 License.
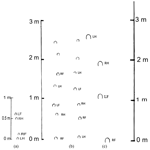
Determining the gait of Miocene, Pliocene, and Pleistocene horses from fossilized trackways
Alan Vincelette
Much work has been done on the study of vertebrate gaits over the past several decades and efforts undertaken to apply this to fossil tracks, especially dinosaurs and mammals such as cats, dogs, camels, and horses. This work seeks to expand upon such studies and in particular to study footprints laid down in sand by modern horses and apply such studies to determine the gaits of fossil horse trackways. It thus builds upon the work of Renders (1984a, b) and Kienapfel et al. (2014) and suggests additional measurements that can be taken on horse footprints. In this study the footprints left in the sand by 15 horses of various breeds with various gaits were videotaped, photographed, described, and measured in order to determine characteristics useful in distinguishing gaits. These results were then applied to two new sets of fossil footprints, those of the middle Miocene merychippine horse Scaphohippus intermontanus that I personally examined and measured and those from the late Pleistocene horse Equus conversidens, previously illustrated and described in the literature (McNeil et al., 2007). The latter horse exhibits a fast gallop of around 9.4 m/s, but it is the former whose footprints are quite unique. The quantitative and visual features of these prints are suggestive of a medium-fast gait involving apparent “understepping” of diagonal couplets and hind feet that overlap the centerline. The gait that most closely matches the footprints of Scaphohippus is the “artificial” gait of a slow rack or tölt, or pace, around 1.9 m/s, though an atypical trot of a horse with major conformation issues or which is weaving (swaying) from side to side is a less likely possibility. This intimates, along with the earlier study of Renders (1984a, b), who found the artificial gait of the running walk displayed by Pliocene hipparionine horses, that ancient horses possessed a much greater variety of gaits than modern horses and that over time they lost these abilities with the exception of certain gaited breeds.
- Article
(1990 KB) -
Supplement
(976 KB) - BibTeX
- EndNote
Among horses, one of the most diverse sets of gaits of any animal species can be observed. Some of these gaits are standardly classified as “natural” as they occur in nearly all horse breeds and usually do not have to be explicitly taught to the horses. There are four such natural gaits: the walk, trot, canter, and gallop, in increasing order of speed (Grogan, 1951; Hildebrand, 1965, 1977; Gray, 1968; Prost, 1970; Leach and Dagg, 1983; Barrey, 1999, 2013b; Starke et al., 2009; Harris, 2016, pp. 55–93; Serra Bragança, 2020, 2021). Other horse gaits are termed “artificial”, “acquired”, “ambling”, or “easy” as they tend to be restricted to specialized breeds and may require extensive training of the horse to bring them out, yet are quite smooth for the rider. These artificial gaits include the paso fino, running walk, fox trot, tölt, and pace (Nicodemus and Clayton, 2003; Ziegler, 2005; Robilliard et al., 2007; Harris, 2016, pp. 95–122). It should be noted, however, that the word “artificial” is here somewhat of a misnomer as many of these gaits appear spontaneously in different horse blood lines and may also be exhibited in foals of multiple horse breeds before being lost or untrained.
Horse gaits can also be distinguished by whether they are symmetrical, that is the legs mirror each other on both sides of the horse's body; are coordinated; and so involve ipsilateral or contralateral limbs moving together (i.e., lateral or diagonal coordination) as opposed to involving limbs that move individually or in a dissociated manner as well as in terms of number of beats, speed, stride or cycle length, overtracking (overstepping), duty factor, suspension phases, and support structures, along with other characteristics such as advanced placement. Many studies have been devoted to describing the nature of such gaits. I collate and reproduce the key findings of these studies below (see also Tables 1 and 2).
1.1 Symmetrical laterally dissociated gaits
Symmetrical laterally dissociated gaits are those wherein the limbs chiefly operate independently of each other, beginning with a sequence of limbs moving one after the other on one side of the horse followed by a similar sequence on the horse's other side (hence such gaits have sometimes been described as bipedal or square). This almost always involves a footfall pattern of left hind (LH), left front (LF), right hind (RH), and right front (RF). Such gaits include the walk, paso fino, and running walk of horses.
The walk is the natural slow-speed gait of the horse. It occurs at a typical speed of 1.4–1.8 m/s and with a cycle (stride) length of 157–193 cm. Because it involves laterally dissociated and isochronous limb movements, it is a four-beat gait. It is also a non-suspended gait as there is at least one foot on the ground at all times. In fact in the walk there are typically two or three limbs supporting the horse's weight at all times, or more particularly a triangular support structure followed by alternating lateral and diagonal bases of support. This makes the walk quite stable and easy on the horse. More technically the walk has a duty factor around 0.6 as each limb is on the ground for around 60 % of the stride and a stride frequency of around 0.8–1.1 strides per second depending upon the speed. The walk can occur at slow (1.2–1.3 m/s), medium (1.3–2.2 m/s), or fast (2.2–3.0 m/s) rates. Horses can also engage in a collected walk with short, quick, and elevated marching steps around 150–160 cm in length or an extended walk with longer and less frequent steps around 180–200 cm in length. In slow and collected walks the three-legged support structure dominates, and the hind feet tend to make contact with the ground behind (undertracking) or overlapping (capping) the ipsilateral front feet. In medium-speed (working) walks the hind feet typically contact the ground just in front of the ipsilateral front feet. Finally, in extended and fast walks the hind hooves often overtrack (overstep) the ipsilateral front hooves. The walk will normally transition to the trot at high speeds (Clayton, 1995; Nicodemus and Slater, 2009; Weishaupt et al., 2010; Solé, et al., 2013).
There also exist some “artificial” laterally dissociated gaits found in specialized horse breeds. These artificial gaits, like the standard walk, are isochronal four-beat symmetrical gaits and display the same footfall sequence of left hind, left front, right hind, and right front but are modified in various ways, most often so that the gait is smoother for the rider.
The paso fino of Columbian Paso horses is a slow (0.9–1.3 m/s) walking gait but one that is highly collected with high and quick steps (stride frequency around 2.6 strides/s), one possessing a duty factor of 0.55. In the paso fino the hind feet will usually undertrack the ipsilateral front feet by a considerable distance, and so contralateral feet come down near each other, forming diagonal couplets. As it is slow and collected, there are always at least three feet on the ground for increased support and a comfortable gait that can be sustained for a long time (Nicodemus and Clayton, 2003; Novoa-Bravo et al., 2018).
The running walk (slow amble, paso llano) is the artificial medium to fast gait of Tennessee Walking, Spotted Saddle, and Peruvian Paso horses. The running walk can occur at medium (ca. 2.7 m/s) to fast (ca. 3.8–4.1 m/s) speeds. It possesses an extended cycle length wherein the hind feet overtrack the ipsilateral front feet by several inches, forming lateral couplets at medium speeds, four individual imprints at fast speeds, and diagonal couplets at very fast speeds as the overstep exceeds 25 % of the cycle length. This results in the limbs being on the ground 40 % to 50 % of the stride cycle (i.e., a duty factor of 0.4 to 0.5) and the body being occasionally supported by only one limb as opposed to the continual two or three limb support in the standard walk. Still as the support structure in the running walk typically involves an ipsilateral or diagonal two-limb support structure, along with a brief tripedal support period, it is a smooth gait for the rider (Renders, 1984a, b; Nicodemus et al., 2002). Tennessee Walking and Missouri Foxtrotting horses can also exhibit a slower variation in the running walk, the flat walk, again with extended strides and overtracking but at a speed of only 2–3 m/s.
1.2 Symmetrical diagonally coupled gaits
The trot is the natural medium to fast gait of the horse, which is an isochronal diagonally coupled two-beat gait wherein contralateral front and hind limbs move in a coordinated manner, lifting off the ground in sync, swinging together, and striking the ground nearly at the same time (the hindfoot sometimes contacts the ground just before the front foot or vice versa), forming lateral couplets. The two-beat footfall sequence of the trot is hence LH + RF − RH + LF. This results in two suspended phases in the stride wherein all four feet are off the ground (for 9 % of stride duration). The trot has a typical duty factor of 0.35–0.45 and a frequency of 1.5–2.0 strides/s. Like the walk, the standard trot can occur at different speeds: slow (jog), at speeds of 2.5–3.6 m/s with moderate undertracking; medium, at speeds of 3.6–5.4 m/s with capping of hoofs; or fast, at speeds of 5.4–9.0 m/s with overtracking of front feet by hind feet. The cycle (stride) length of the trot is typically around 200–450 cm. The trot may also be collected or extended. The collected trot employs short, high-stepping, and quick strides and often undertracks as in Hackney and Morgan horses and can have a duty factor up to 0.6. The extended trot is a fast trot with long strides, increased suspension phases, and a great deal of overtracking (Streitlein and Preuschoft, 1987; Clayton, 1994; Holmström et al., 1994; Leleu et al., 2004; Weishaupt et al., 2010; Hobbs et al., 2016; Walker et al., 2017). The Standardbred horse can perform an extremely fast flying trot at speeds of 10.0–14.2 m/s, a stride frequency of 2.5 strides/s, and a cycle length of 500–600 cm. The flying-trot beats are asynchronous (irregular), with an abaxial lateral offset of the hind limbs, and turns into a four-beat gait (Jordan, 1910; Drevemo et al., 1980a, b). The trot can rapidly cover a fair amount of ground, yet it gives the horse a solid diagonal base of support between suspended phases, thus providing lots of balance for uneven surfaces. The horse, moreover, is capable of maintaining the trot for a long time. The trot is less comfortable for the rider, however, as the horse bounces up and down.
There are a few “artificial” diagonally coupled gaits. The passage (prancing trot) is a slow, collected, elevated trot found in American Saddlebred, Andalusian, and Lippizan horses as well as in and Dutch, German, and Swedish Warmbloods and indeed is considered a demonstratable natural gait in the German riding tradition. It is a two-beat gait, but in the passage the stride becomes slightly asynchronous, with the hindfoot touching down just before the diagonal front foot, resulting in a brief period of single-digit support between the diagonal phases and extended periods of floating suspension. The duty factor of the passage is around 0.4. The passage has a smoother ride than the trot but is much slower (1.2–1.8 m/s) and tiring for the horse. These same horse breeds can also perform the piaffe, which is an even more collected, elevated, and slower gait, with a very short stride, and the horse almost appears to trot in place. The piaffe has a duty factor between 0.45–0.5. There is no suspension phase at all in the piaffe, and the support structure varies between two diagonal to three limbs on the ground (Weishaupt et al., 2009; Clayton and Hobbs, 2019).
The fox trot (pasitrote) is another diagonally coupled gait found in the Missouri Foxtrotter and the Kentucky Mountain horse. It is a medium-slow speed trot involving four highly asynchronous (irregular) beats, short quick steps (1.6–2.3 strides/s in the fox trot, up to 2.7–2.9 in the trocha), diagonal couplets, and undertracking to slight overtracking. The speed of the fox trot is around 3–4 m/s, with strides around 200 cm long. The fox trot is the trot-based equivalent of the slow gait or broken pace. That is to say the fox trot is a broken trot wherein diagonal limb pairs lift off the ground at the same time and swing forward together, but the front foot strikes the ground slightly before the contralateral hind foot (by 10 %–15 % of stride duration), resulting in a slight delay between the striking of ipsilateral feet and a footfall pattern of LH − (LF − RH) − RF. For this reason the fox trot is sometimes described as a horse walking with its front legs while trotting with its hind legs. In the fox trot there are two alternating lateral and diagonal feet on the ground for support for much of the stride (82 % of stride) and sometimes three feet (18 % of stride), with a duty factor of 0.5 and no aerial phase. It is smooth for the rider and stable and easy for the horse, although there is a slight rocking back-and-forth motion. The Carolina Marsh Tacky, the Columbian Paso Fino, and the Mangalarga Marchador can also perform a slow collected fox trot (the trocha or marcha batida) wherein the support structure varies between two diagonal limbs to three or even four limbs on the ground at once (Clayton and Bradbury, 1995; Nicodemus and Clayton, 2003; Nicodemus and Slater, 2009).
1.3 Symmetrical laterally coupled gaits
Not surprisingly, all of the laterally coupled gaits are “artificial” as they involve the horse balancing its weight on one side while the ipsilateral limbs on the other side move in conjunction. The slow gait (stepping pace, paso corto, sobreandando, marcha picada) is a medium-slow-speed gait (2.0–3.5 m/s) found in American Saddlebred, Paso, and Mangalarga Marchador horses. The slow gait has a typical stride duration of 0.6–0.5 s, but the marcha picada variation found in the Mangalarga Marchador has a larger stride duration of 0.9 s. It has four beats along with a collected, quickened, and often heightened stride with a fair amount of undertracking. In the slow gait the ipsilateral hind and fore feet start the gait at the same time, but the hind foot contacts the ground slightly before the ipsilateral front foot. This causes the gait to become asynchronous with a slight delay between the second and third beats as the lateral leg pairs strike the ground closer together than the diagonal leg pairs. The slow gait hence has a footfall pattern of (LH − LF) − (RH − RF). The slow gait is thus a sort of broken pace, with the lateral feet taking off together but landing separately in order to eliminate the suspension phases, which is why it is also called the stepping pace. In the slow gait, accordingly, there is only a brief period where there is just one foot on the ground, and normally two and sometimes three feet make contact, making it a smooth gait to ride, one that can cover ground more quickly than the walk but without the vertical motion of the trot or the rolling motion of the pace. It is also similar to a slow rack but with the slight delay between the second and third beats. Though not overly tiring for the horse and capable of long distances, the slow gait is hard on the horse's joints (Gonçalves Fonseca et al., 2018).
The rack (amble, tölt, paso largo, huschano, trippel, revaal) is a slow to medium-fast artificial gait found in American Saddlebred and Standardbred horses; Rocky Mountain and Florida Cracker horses; Paso horses; the Icelandic horse; and Indian Marwari, Kathiawari, and Sindhi horses. The rack or tölt has the same general footfall pattern as the walk, (LH − LF) − (RH − RF), with equal intervals between each of the four beats and a duty factor of 0.35–0.55, but it has higher and quicker strides (1.5–2.2 strides/s) and more extended steps, with cycle lengths around 150–300 cm long. The rack is a sort of broken pace wherein the hind and front hooves lift off the ground almost simultaneously, but the hind hoof comes back down a little before the ipsilateral front hoof. At slower speeds of 3–6 m/s (walking rack, pleasure rack, stepping rack), the rack has the same support structure as the walk, with alternating lateral and diagonal limb pairs followed by three limbs on the ground. As there is only a moderate amount of overstepping, ipsilateral couplets tend to be present. At fast speeds of 6–9 m/s there are periods when there is just one foot on the ground (hence the alternative names of (single-foot, coon rack, or speed rack), followed by alternating lateral and diagonal support phases with two feet along with lots of overtracking. This results in diagonal couplets forming as contralateral limbs land close together. The rack is comfortable for riders, but it is quite tiring for horses and cannot be maintained for long periods of time (Zips et al., 2001; Nicodemus and Clayton, 2003; Pecha et al., 2011; Boehart et al., 2013; Waldern et al., 2015; Gunnarsson et al., 2017; Stefánsdóttir et al., 2021).
The fastest laterally coupled gait is the pace (huachano, Schweinepass, fast amble), which is a two-beat gait found in Standardbred, Peruvian Paso, and Icelandic horses. The pace is even faster than the trot as it involves diagonal couplets and less interference of limbs and is capable of speeds of 9.0–16.0 m/s, though it most often occurs at around 5–8 m/s. In the pace, though the lateral limbs lift off in unison and swing forward together, the hind foot often makes contact just before the front foot, with each limb remaining on the ground for 28 %–35 % of the gait. The pace also has a very high stride frequency of 1.8–2.7 strides/second, and its length is typically between 300–600 cm. The footfall sequence in the pace is LH + LF − RH + RF, and it has a support structure of opposing ipsilateral pairs. The pace can be performed in a slow and collected manner (pacing walk) where undertracking often occurs, and one forelimb may contact the ground before the contralateral limb lifts off, resulting in brief diagonal support phases alternating with lateral support phases and the suspended phases being reduced or eliminated. Icelandic horses display a very fast variant called the flying pace (skeið), which can achieve speeds of up to 13.0 m/s for short durations, with a large amount of overtracking (over 25 % of the cycle length) and couplets that are now lateral ones. The pace is not as stable and balanced as the trot but is easier on the horse and more comfortable for the rider, albeit producing a side-to-side rolling motion as well as a fore and aft see-sawing motion (Wilson et al., 1988a; Boehart et al., 2013; Stefánsdóttir et al., 2021).
1.4 Asymmetrical gaits
Horses possess two main asymmetrical gaits with limb movements that do not mirror each other on the two sides of the horse's body and so what can be described as a leading foot. These are also fast three- to four-beat gaits, typically with only a single suspension phase and naturally occurring in horses. At medium-fast speeds horses often engage in the three-beat canter (Canterbury gallop), wherein diagonal limbs impact together for the second beat. The canter is particularly prominent in the American Saddlebred, Tennessee Walking, and Western Pleasure horses; Dutch, German, and Swedish Warmbloods; Icelandic horses; and the Mangalarga Marchador. The canter typically occurs at a medium-fast speed (4–8 m/s) during which the horse moves forward in a slightly diagonal direction. The canter has a cycle frequency of 1.5–2.5 cycles per second, a duty factor of 0.35, and a cycle length of around 200–400 cm. If the horse canters with a left lead then the sequence of the three beats is right hind, the diagonal left hind and right front feet spring and land together (or on occasion the hind foot lands just before the front foot), and finally the left-leading forelimb hits the ground, forming a footfall pattern of RH − LH + RF − LF. If the horse canters with a right lead then the sequence of the three beats is left hind, then the diagonal pair of the right hind and left front move and touch down together, and finally the right forelimb makes contact, so it has a footfall pattern of LH − RH + LF − RF. In the standard canter the support structure cycles between one and two diagonal limbs on the ground followed by an aerial phase. In the slow collected canter (“slow gallop”) of the Columbian Paso horse, the horse takes short and elevated steps, and at least one limb is on the ground at all times, with the hindfoot undertracking the ipsilateral front foot. In the medium-speed collected canter (or lope in western riding) with a speed around 1.8 m/s, a cycle frequency of 1.4 cycles/s, and a cycle length around 130 cm, there are occasions where all three limbs are on the ground at once, but there is also an aerial phase. The canter is less tiring for the horse than the gallop and can provide good diagonal or triangular support on rough terrain, but it is not as fast as the trot or gallop (Deuel and Park, 1990; Loitsch, 1993; Clayton, 1993, 1994; Back et al., 1997; Nicodemus and Clayton, 2001; Nicodemus and Booker, 2007; Splan and Hunter, 2004).
At fast speeds the horse naturally transitions to a transverse gallop, a fast four-beat gait wherein each limb only makes contact with the ground for 20 %–30 % of the cycle duration. The gallop involves either a left- or right-leading foot. In the left-lead gallop the right hind foot moves first, followed by the left hind and right front legs moving forward in diagonal unison but with the hind limb hitting the ground slightly before the front limb, ending with the independent “leading” left front foot contacting the ground. Hence the footfall pattern of the left-lead gallop is RH − (LH − RF) − LF. In the right-lead version the left hind limb moves first, then the right hind and left front diagonal pair moving forward together with the hind limb hitting the ground slightly before the forelimb, and finally the “leading” right front leg lands, making the footfall pattern LH − (RH − LF) − RF. There is a support sequence of one (54 % of the cycle duration) or two contralateral limbs (27 % of the cycle duration) for much of the gallop cycle as well as an aerial phase (19 %–28 % of the cycle duration). The standard gallop reaches speeds between 9–12 m/s (Bertram and Gutmann, 2009). The cycle length of such a gallop is quite long, varying from 300–500 cm, and the frequency ranges from 2.0–2.9 cycles/s. The fast extended gallop (or run) of Thoroughbreds and Quarter horses can reach speeds of 10–18 m/s, with a cycle frequency of 2.3–3.0 cycles/s, a stance duration approaching 0.1, and a cycle length of 440–700 cm. Though the gallop can attain high speeds, it is fatiguing for the horse and can only be kept up for 3 to 5 km (Deuel and Lawrence, 1986; Leach et al., 1987; Seder and Vickery, 2003a, b; Witte et al., 2006; Takahashi et al., 2007; Parsons et al., 2008; Morrice-West et al., 2020). Horses have also been observed to perform a rotary gallop for short bursts, especially during the initial acceleration phase of a race before switching to a transverse gallop. The rotary gallop is a very fast four-beat, left- or right-lead, double-suspension gait, wherein the limbs alternate between an extended suspension and a collected suspension, causing the pattern of limb impacts to switch diagonally or transversely from right hind followed by left hind to left front followed by right front, i.e., with a footfall sequence of LH − RH − RF − LF (Hildebrand, 1980; Seder and Vickery, 2003a, b; Bertram and Gutmann, 2009; Biancardi and Minetti, 2012).
A 15 m×5 m straight section of track was raked in a sandy horse arena to allow footprints to distinctly show up when horses treaded over it. Fifteen horses of different breeds and sizes (see Table S1 in the Supplement) were ridden or walked over the prepared trackway in various gaits. Breeds studied included Andalusian, Arabian, Quarter horses as well as Saddlebreds, Tennessee Walkers, and Thoroughbreds.
Sketches were made, and photographs taken of the horse footprint trackways (along with videos of the most recently studied horses as indicated in the footnote to Table S1) in order to identify footfall patterns and for rough measurements of speed and distance traversed. Horizontal measurements along the plane of motion were then taken with a ruler or tape measure between the footprints of the gait in accordance with fairly standard protocols, such as measuring the cycle (stride) length, the distance between steps or between front and hind feet pairings (which I have called the intercouplet distance), and the overtrack or undertrack between ipsilateral or diagonal feet pairings (see Leach et al., 1984; Renders, 1984a, b; Thompson et al., 2007). In addition three lateral measurements were taken: the distance ipsilateral (or diagonal) feet pairs were out of parallel alignment (what I have called the lateral offset), the distance between contralateral feet impressions (what I have called the interior straddle in contrast with the standard straddle measurement between outer edges of the hoofs of contralateral impressions or the diagonal width measurement between contralateral mid-hooves as in Leach et al., 1984, fig. 5), and the distance between the center of each ipsilateral or diagonal pairs of feet (which I have called the cross-measure as it often occurs at an angle to the center plane of motion if there is any lateral offset of the couplets; see Fig. 1 below).
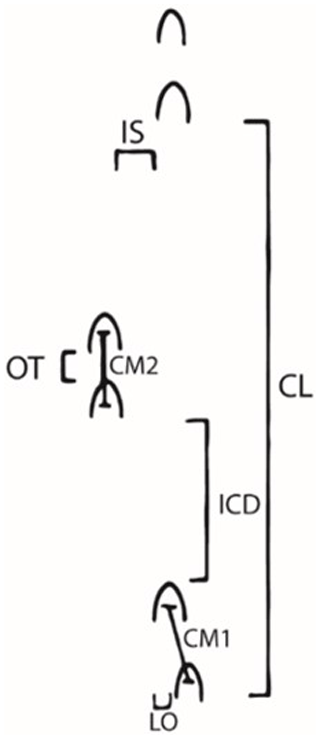
Figure 1Measurements taken on horse tracks. CL: cycle length; ICD: intercouplet distance (i.e., distance between couplets or nearby pairs of prints); OT: overtrack; IS: interior straddle (i.e., between inner edges of contralateral limbs); CM: cross-measure (i.e., distance from the center of one hoof impression to another within a couplet); LO: lateral offset (i.e., lateral distance between edges of footprints in a couplet).
Because access to easy-gaited horses in the western United States is limited, and especially so due to travel and visitation restrictions due to Covid-19, I supplemented the previous data with data culled from videos of horses displaying various gaits. I have kept these data segregated as they are less accurate than the above. I have also made use of the data present in other studies on horse gaits, in particular those of Kienapfel et al. (2014) or Renders (1984a, b). In the data tables given below and in the text, the superscripts V for videos, K for Kienapfel, or R for Renders are attached to a horse number or a measured value to indicate its source, i.e., 18V, 0.12R, and 22K.
Finally the average for each of the measurements was calculated for the distinct gaits and key ratios determined including such ratios as cycle (stride) length height, overtrack intercouplet distance, and cross-measure cycle length (see Tables 3 and 4).
Next sketches were made and measurements taken of two sets of fossil horse footprints (see Tables 5 and 6). The former is a track likely made by the merychippine horse Scaphohippus intermontanus1 around 14.5 Ma in middle Miocene (middle Barstovian Ba2, Barstow Formation) lacustrine sediments of the Mud Hills deposit (Greer Quarry) near Barstow, California. This horse laid down a set of four imprints on a track 61 cm long. The uppermost impression is partial only (reconstructed as a whole print in Fig. 5, with a dotted line indicating where the break occurs). Sarjeant and Reynolds (1999, pp. 15–17), individually described, measured, and photographed these prints (ichnospecies Hippipeda araiochelata), and I too have examined, measured, and traced these footprints and molds of them made in situ at the San Bernardino County Museum in additional ways following the protocol given above.
The latter is a set of footprints made by the late Pleistocene horse Equus conversidens in aeolian sands and silts near a river at the Wally's Beach deposit near Cardston in Alberta, Canada. The trackway consists of five imprints laid down across 401 cm of sand some 11 000 years ago. I, however, here rely upon the descriptions (ichnospecies Hippipeda cardstoni), to-scale drawings, and measurements given in McNeil et al. (2007, pp. 215–218) and have not examined these footprints in person.
Finally the measurements, key ratios, and patterns of the footprints made by the living and fossil horses were compared in order to see if the gait and speed of the fossil horses that left the prints could be determined.
3.1 Observable and measurable differences between footprint patterns within modern horse gaits
The walk, because it is a slower gait, lays down hoof prints in ipsilateral couplets (2–2) that are close together and typically in parallel alignment, alternating between the left and the right side of the centerline (see Figs. 2, 3a). The strides of the walk are short and about the same distance as the height of the horse at the withers, averaging 147.1 and 177.0 cm in a slow and fast walk (or an average ratio of cycle length height of 0.95 in a slow walk and 1.10 in a fast walk), with a distance between couplets (intercouplet distance) averaging 52.7 cm in a slow walk and 57.2 cm in a fast walk, or around 34 % and 36 % of the horse height and 36 % and 32 % of the cycle length, respectively (see Tables S2 and S3 in the Supplement). In slower walks the hind hoof impression often overlaps or caps the impression made by the ipsilateral front hoof, and there may even be an undertrack of up to 6 cm separating the two impressions (hence the overtrack cycle length ratio averaged −0.07 in the slow walk). In faster walks there is moderate overtracking of the front hoof by the hind hoof by an average distance of 3.0 cm (or an overtrack 0.02 the length of the stride). Because in the walk, ipsilateral feet tend to line up in parallel (average lateral offset of 1.7 cm in the slow and 3.1 cm in the fast walk) and close together (average interior straddle of 3.6 cm in the slow and 1.4 cm in the fast walk), the cross-measure between the hoofprints in a couplet is small, averaging 9.1 and 17.6 cm in the slow and fast walk (or a cross-measure cycle length ratio of 0.06 and 0.10 in the slow and fast walk, respectively). The paso fino has footprints and cycle lengths that resemble a very slow walk.
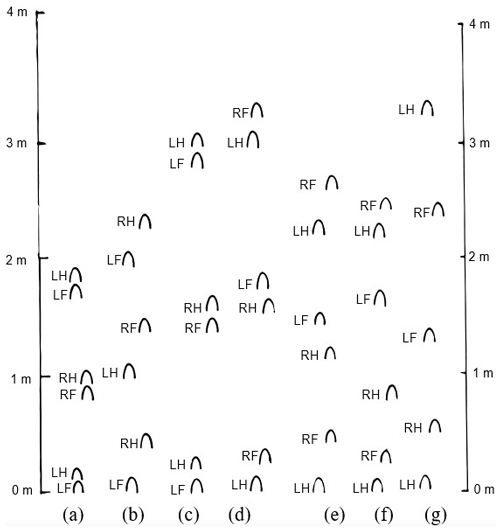
Figure 2Illustrations of tracks left in the sand by various horse gaits. (a) Fast walk, (b) running walk, (c) fast trot, (d) fast rack, (e) slow pace, (f) left-lead canter, (g) right-lead gallop. LH: left hind print; RH: right hind print; LF: left front print; RF: right front print.
The running walk also lays down prints in ipsilateral couplets (2–2) but in a considerably faster gait with a longer cycle length (averaging 1.35 times the horse height). Consequently there is considerable overtracking, on average 24.8 cm (12 % of the cycle length); the intercouplet distance shrinks somewhat to an average of 42.6 cm (20 % of the cycle length); and the overtrack distance between ipsilateral hoof impressions begins to close in on the distance between couplets (the ratio of the overtrack intercouplet distance being 58 %). Generally speaking in the running walk, as in the standard walk, the ipsilateral prints will line up in a parallel line and again just to the left or right of the centerline. However at very fast speeds the trackway of the running walk begins to look like four individual prints spaced roughly equally apart rather than ipsilateral couplets (consult Fig. 2 and Table S4 in the Supplement).
The trot again lays down tracks in ipsilateral couplets with the footprints of the ipsilateral pair occurring close together but not as close as in the standard walk (see Figs. 2, 3b). Because the trot is a faster gait the cycle length is long, averaging 193.9 and 265.1 cm in a slow and fast trot (forming around a 1.23 ratio in relation to horse height in a slow trot and 1.71 in a fast one), and the intercouplet distance is large, measuring 71.0 and 103.0 cm in the slow and fast trot (see Tables S5 and S6 in the Supplement). As a result the intercouplet distance cycle length ratio is just slightly larger than in the walk (0.37 and 0.39) but considerably larger in comparison to horse height (0.45 and 0.66). In slower trots the hind feet usually undertrack and fall short of the ipsilateral front feet by an average of 3.2 cm (−0.06 ratio for overtrack cycle length), but in fast trots there can be a fairly large overtrack of up to 5 cm (but with an average value of −0.002 of the overtrack cycle length ratio as the stride is quite long). The ipsilateral pairs in the trot are also close to the centerline, with the interior straddle averaging 2.8 and 2.3 cm in the slow and fast trot, but there is a greater tendency for ipsilateral feet to be out of parallel alignment (resulting in an average lateral offset of 3.0 and 5.0 cm for the slow and fast trot). As a result, there is an average cross-measure of 15.2 and 13.9 cm in a slow and fast trot (or 8 % and 5 % of the cycle length, respectively). The passage displays footprints resembling a very slow trot (see Fig. 4a).
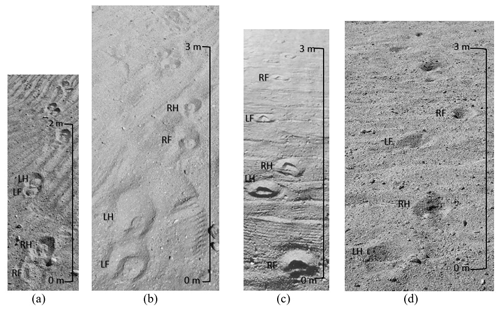
Figure 3Photographs of four common horse gaits. (a) Fast walk (Horse 10a), (b) fast trot (Horse 10b), (c) right-lead canter (Horse 9), (d) right-lead gallop (Horse 8). LH: left hind print, RH: right hind print; LF: left front print; RF: right front print.
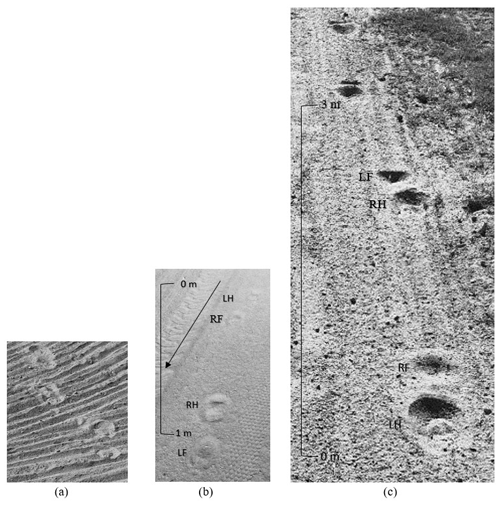
Figure 4Photographs of some “artificial” horse gaits. (a) Passage (Horse 10), (b) slow gait (Horse 15), (c) fast rack (Horse 15). LH: left hind print; RH: right hind print; LF: left front print; RF: right front print.
The medium to fast rack or tölt has a quite different appearance than the standard walk or trot. For in the medium to fast rack (as well as in the pace) the couplets are formed by contralateral feet landing near each other, typically with “undertracking” of the rear print behind its contralateral front partner, and so form pairs that point away from the centerline in alternating directions (see Figs. 2, 4c). This gives rise to a “wave-shaped” trackway of footprints. The rack of the single individual studied here featured a cycle length of 308.9 cm (1.99 times the size of the horse height) and a large intercouplet distance as with the fast trot of 109.9 cm (71 % the size of the horse height and 36 % the size of the cycle length). The rack here featured an undertrack of contralateral feet of 11.4 cm (or 0.09 the length of the long stride). There were two key distinguishing features of the rack (as well as the pace). The first is that as the rack features contralateral couplets there is a large lateral offset averaging 5.1 cm and an even larger cross-measure of 28.3 cm (0.18 the length of the stride). This is even the case in spite of the second key feature of the rack (or pace), a tendency for a very narrow track and contralateral hoof prints that laterally overlap (interior straddle of −6.6 cm). The footprint pattern and measurements of the pace are similar to those of the rack; however, they could only be studied here indirectly via video. In the pace, in contrast to the rack, the hind feet tend to overtrack the contralateral front feet as the gait occurs at high speeds (see Tables S7 and S8 in the Supplement for measurements of the rack (tölt) and pace). The related slow gait visually resembled a slow rack in having a wave-like shape to the footprint pattern as the couplets are comprised of alternating pairs of diagonal feet wherein the hind hoof undertracks the contralateral front hoof but possessed a shorter cycle length and intercouplet distance (see Fig. 4b). The standard fox trot, though not studied in depth here, also lays down footprints similar to a slow rack as even though the limbs are diagonally coordinated at its slow speed, diagonal limbs strike the ground near each other, forming diagonal couplets.
The canter is visually unmistakable as it lays down a track with a 1–1–2 (or technically 1–2–1) sequence of prints, typically with two medium-length steps of nearly equal distance after the couplet, followed by a step of longer or shorter distance depending upon the speed (see Figs. 2, 3c). In terms of measurables, the canter displays a long stride that averaged 252.1 cm (1.60 times the height of the horse). Contralateral feet usually fall near the centerline (average interior straddle of 1.8 cm), but as the couplet is formed by the footfalls of contralateral pairs it is quite extensive here, as in the rack, averaging 5.9 cm and forming a couplet angling away from the centerline to the left or right, depending upon the lead. The horse also tends to move in an overall diagonal in the canter (see Table S9 in the Supplement).
The gallop is also easy to recognize as it consists of four individually separated prints with long distances between them (see Figs. 2, 3d). The first two prints tend to be closer together (28.4 cm apart on average), followed by three longer steps which are on average 73.5 cm long. This gives rise to a long stride of around 307.0 cm, or almost double the size of the horse height (1.91 on average), in just a restrained gallop. In the gallop the contralateral footprints fell farther away from the centerline than in the other gaits, with an interior straddle averaging 9.2 cm (see Table S10 in the Supplement).
3.2 Description and measurements of fossil horse tracks
In addition to studies undertaken on the modern horse tracks noted above, illustrations and measurements were also taken of the two sets of fossil horse tracks described above.
The foot impressions left by the middle Miocene merychippine horse Scaphohippus intermontanus around 14.5 Ma are unusual in appearance (see Fig. 5 below). Four imprints occur in two couplets that overlap the centerline (interior straddle of −1.0 cm) and alternate in pointing outward to the left and then to the right. The uppermost impression is partial only as noted earlier. The three complete foot impressions measure 5.2, 5.0, and 4.7 cm in length and the cracked impression 1.7 cm. The width of the three complete prints is 3.5 cm, while the width of the incomplete impression is 3.7 cm. These measurements were taken on the mold of the prints in order to capture the distances between the prints. The prints themselves are slightly larger, having been measured as 6.2, 5.6, and 6.2 cm in length and 3.8 cm in width (Sarjeant and Reynolds, 1999, p. 15), and shown to be equivalent in size to adult hoof bones assigned to the same species found nearby. Because front feet of horses are often shorter and wider than hind feet (Stachurska et al., 2008; Reynolds, 2006), this suggests that there is considerable undertracking displayed here, namely ca. −10.9 cm (5.3–5.8 cm between prints within a couplet), or −0.11 the size of the cycle length. There is also a large amount of lateral displacement, 1.8 cm in the lower couplet and 1.3 cm in the upper couplet (for an average of 1.6 cm, or 0.02 the size of the cycle length), and a sizable average cross-measure of around 11.1 cm, or 0.11 the size of the cycle length.
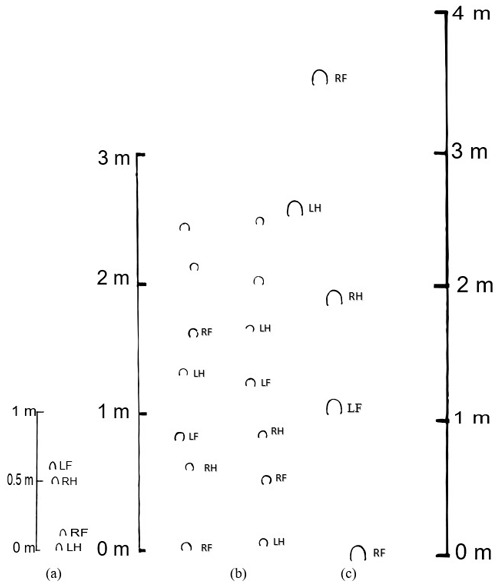
Figure 5Illustrations of fossil horse tracks. (a) Merychippine horse tracks (Scaphohippus intermontanus). Dotted line in foremost Scaphohippus print indicates where impression is broken off. (b) Hipparionine horse tracks (Eurygnathohippus hasumense) after Renders (1984a, b, fig. 2), (c) Equus conversidens tracks after McNeil et al. (2007, fig. 16). LH: left hind print; RH: right hind print; LF: left front print; RF: right front print.
The fossilized track made by the late Pleistocene horse Equus conversidens (McNeil et al., 2007, fig. 16) consists of five hoof imprints 10.5 cm in length and width. The distance between the steps based upon the scale drawing were 100, 70.9, 56.4, and 87.3 cm proceeding from start to end of the cycle. The interior straddle varied from 3.6–12.7 cm.
To determine the other key ratios related to horse footprints the size of the horse at the withers needs to be determined. Studies have shown correlations between adult horse size and hoof size (Stachurska et al., 2011), third metacarpal length (Onar et al., 2018), and cranial length along with third metapodial length (Chrószcz et al., 2014). As the remains of the horses studied here involve footprint impressions, which from my observations are nearly identical to horse hoof size in slower gaits, utilizing the correlations of hoof size with height at the withers is an important starting point. Stachurska et al. (2011) found the height at the withers in Arabian and Half-Arabian horses to be 12.83–13.16 times the size of the front hoof length. This would yield a height of ca. 72.8 cm for Scaphohippus intermontanus, which had a front hoof 5.6 cm long, and a height of 136.4 cm for Equus conversidens, which had a front hoof 10.5 cm long. In addition, if we use the cranial length of 31.5 cm for a Scaphohippus intermontanus skull of ca. 14.9 Ma (American Museum of Natural History, AMNH: FM 87301) and multiply it by the factor of 2.7 suggested by Chrószcz et al. (2014, p. 145), we get a height of 85.1 cm.
That being said, studies have suggested that metacarpal size is a more reliable predictor of overall horse size than either cranial or phalanx length (Alberdi et al., 1995; Chrószcz et al., 2014). Hence it is important to also utilize the study of Onar et al. (2018, p. 163), who found that the height of modern horses averaged 5.66 times the size of the metacarpal length. Postcranial remains of Scaphohippus intermontanus are relatively rare, but Merriam (1919, pp. 502–503) assigns one complete metacarpal found in nearby strata to the species. It measured 16.5 cm (the metatarsal was 18.1 cm long). This would yield a height of 93.4 cm. Equus conversidens has a metacarpal that averaged 22.8 cm long (Harris and Porter, 1980, p. 50), which would yield a height of 129.0 cm.
In what follows I estimate the height of the fossil horses by averaging these two values, i.e., the estimation of size based upon hoof length and metacarpal length. This yields a height of 79.2 cm for Scaphohippus intermontanus and 132.8 cm for Equus conversidens.
Finally, I wanted to include footprints of the hipparionine2 horse Eurygnathohippus previously studied by Renders (1984a, b). Renders (1984a, p. 179) records a hoof length of 6.5 cm, which would yield a height of 84.4 cm per the above formula of Stachurska. Hooijer (1975, p. 33) records the length of a skull (BK II, pit 6 (upper Bed II), nos. 2845–2846) of 26.0 cm, which would yield a horse height of 70.2 cm. Bernor et al. (2005, pp. 152 and 157; AL 155–6) and Van der Made (2014, fig. 8) record the size of the third metacarpals of Hipparion (Eurygnathohippus) as 26.2 and 24.0 cm. This would give a horse height of 142.1 cm according to the formula above. If we average the value from the hoof and metacarpal lengths we get an estimated height of 105.9 cm.
Scientific inquiry into the gaits of extinct vertebrates has increased exponentially over the past few decades. Extensive investigations have been undertaken on the gaits of dinosaurs (Alexander, 1976; Thulborn, 1982, 1989; Mazzetta and Blanco, 2001; Henderson, 2006; Stevens et al., 2016; Bordy et al., 2020), camels (Alf, 1966; Webb, 1972; Sarjeant and Reynolds, 1999; McNeil et al., 2007; Thompson et al., 2007; Cabral-Perdomo et al., 2018), and horses (Renders, 1984a, b; Kienapfel et al., 2014), among other vertebrates. Such studies have compared cycle (stride) length, speed, and footprint patterns of living species with fossilized trackways in order to elucidate the gaits of extinct vertebrate species.
This paper expands upon such work and examines footprint patterns of several living horse breeds in order to increase the database of gaits in living analogs. In particular extensive measurements have been taken on various facets of horse footprints, including cycle (stride) length, overtracking, distance between couplets (intercouplet distance), distance between contralateral feet (interior straddle), and the alignment of prints in a couplet (lateral offset and cross-measure), in order to distinguish various horse gaits. It then applies the results to two sets of isolated, extensive, and clearly defined fossil horse tracks, one from the merychippine horse Scaphohippus intermontanus in middle Miocene strata from the Mud Hills deposits of California and another from Equus conversidens in late Pleistocene rocks from Wally's Beach deposits in Alberta, Canada, in order to determine the gaits exhibited. Finally a reevaluation of the tracks of the hipparionine horse Eurygnathohippus from Pliocene rocks at site G of the upper Laetoli Beds in Tanzania (Renders, 1984a, b) is undertaken.
The largest and most recent horse, the late Pleistocene Equus, exhibited a left-lead gallop at a fairly rapid speed of 9.4 m/s. Such a gait is evident as the footprint pattern shows four separate prints nearly equidistant apart and spaced out over a long stride. The high ratio of stride height of 2.69 is indeed indicative of a very rapid gallop. It is hard to know why this Pleistocene horse was running at such a fast speed; perhaps it was being chased by predators, migrating, or just out for fun.
Intriguingly, the data suggest that Scaphohippus intermontanus was displaying the “artificial gait” of a slow rack or tölt (or slow pace or slow gait) of around 1.9 m/s. The ratio of the stride length height of 1.18 suggests a medium-speed gait such as a slow trot or rack, as do the values of 0.42 and 0.34 for the intercouplet distance height ratio and −0.33 and −0.11 for the overtrack intercouplet distance and overtrack cycle length ratios. Three factors, however, point to this gait being a rack or tölt (or slow gait, fox trot, or slow pace) as opposed to a trot. In the first place the footprints display a considerable negative interior straddle of around −1.0 cm. A negative interior straddle was most often seen occurring in diagonally coupled gaits as there is room for the limbs to come close together without interference. In fact negative interior straddles seem relatively rare in the trot, though they can occur on occasion (I observed one such occurrence in horse 3 of −5.1 cm), usually due to conformation issues or a horse that is weaving (swaying) from side to side rather than moving in a straight line; see Figs. S1 and S2 in the Supplement and Kienapfel, 2014, figs. 6 and 7). Second, in spite of the negative interior straddle there are fairly large cross-measure height and cross-measure cycle length ratios found in the tracks of Scaphohippus here, namely values of 0.14 and 0.11. Now the average values of cross-measure height and cross-measure cycle length ratios are 0.22 and 0.8 in a slow trot versus 0.18 and 0.11 in a slow rack. This again is suggestive of a rack being exhibited by Scaphohippus; however, the occasional slow trot can have a cross-measure cycle length ratio of 0.12 (as happened once in horse 9). Finally, and perhaps most importantly, the Scaphohippus footprints suggest an “undertracking” of diagonal couplets as the nearby print pairs are laterally offset while forming a line that points away from the centerline of the stride, or in other words forming a wave-shaped trackway (see Figs. 5 and 6). An occasional trot, wherein the horse has poor conformation and is dishing or plaiting or where it is weaving (swaying) from side to side, can also form a track with a wave shape (as happened in horse 3; see Fig. 6 below and Figs. S1 and S2 in the Supplement), but individual hoof impressions will usually point towards or away from the centerline and not line up perpendicular to the centerline as is the case in the fossil footprints studied here. So the fact that here the hoof impressions all individually orient in a forward-pointing manner but that the hind feet overlap and alternate between being to the left and then to the right of the front foot strongly suggests a gait with diagonal limbs landing near, as found in the rack and the pace (as well as the slow gait and fox trot). Indeed in a rack with “undertracking” (understepping) the hind feet (here at the rear of the couplets) tend to align with each other as they transition to an inner track and straddle the centerline, whereas in a wave-shaped trot with undertracking it is most often the innermost prints formed by the ipsilateral feet that align in the two couplets (see Fig. 4b; see also Kienapfel, 2004, figs. 5, 6, 7). Such alignment of the hind feet is found within the Scaphohippus prints. Hence if we superimpose the Scaphohippus prints over rack and wave-shaped trot trackways, the fit is better for the rack than the wave-shaped trot (see Fig. 6). All in all then, the gait that best fits the measurements and patterns displayed by the Scaphohippus prints is a slow to medium rack with undertracking of contralateral feet, though a trot is not outside the realm of possibility. It is unfortunate that only four impressions were preserved as a few more impressions could have provided additional evidence as to which gait was occurring in Scaphohippus.
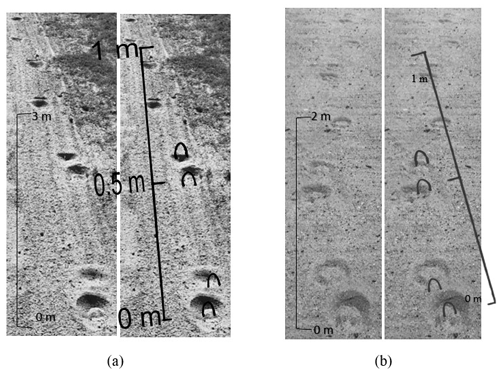
Figure 6(a) Scaphohippus tracks scaled up, rotated, and superimposed over rack trackway of Horse 15 to produce best possible fit; (b) Scaphohippus tracks scaled up, rotated, reversed, and superimposed over wave-shaped trot trackway of Horse 3 to produce best possible fit. Note better overall alignment with rack than with trot; how the fossil back prints line up nearly in parallel as in the rack as opposed to the trot; and that in the wave-shaped trot displayed here the modern horse front prints are pointing away from centerline but not in the fossil prints, which occurs when an irregular-shaped trot is formed.
If Scaphohippus was indeed engaging in the “artificial” gait of a rack or tölt, or a pace, then it seems earlier horses were quite “gaited” and had laterally coupled gaits naturally available to them. Renders (1984a, b) has already shown that Eurygnathohippus horses could stride in a running walk gait, which is not a natural gait of horses. There could be several explanations for why this horse was engaging in a rack or slow pace. A pacing gait is sometimes seen in animals that are juveniles, weak, or fatigued. Hence some foals pace before learning how to trot. Still the horse tracks of Scaphohippus match the size of adult coffin bones, and so they do not seem to be that of an overly young horse. Such alternative gaits could have been useful to early horses as they allow horses to travel at a similar or faster speed than in the trot but with less expenditure of energy. Such gaits, though not as stable as the trot, may additionally have been useful in allowing fast speeds over varied or uneven terrain for they allow the horse to switch between a lateral and diagonal limb support structure and keep one foot on the ground at all times. This is one reason the tölt is useful in Iceland, with its varied volcanic and glacial outcroppings, and why the pace is beneficial in camels on the sand dunes. Another possibility is that Scaphohippus was an early grazing horse (Feranec and Pagnac, 2017), and it may have quickly developed long legs but with a relatively short body and hence found the rack or slow pace more efficient or a way to avoid limb interference as in camels or dogs.
Renders (1984a, p. 179), in fact, has already used comparisons between living horse footprints and those displayed by two adult hipparionine horses (Eurygnathohippus hasumense) at site G of the upper Laetoli Beds in Tanzania to argue for their traveling in the “artificial” gait of a running walk of 1.8 and 2.2 m/s. I concur with her assessment, though I have recalculated the speeds of the two gaits as even faster, at 2.2 and 2.4 m/s, based upon newer estimates of horse height. I should also note that Renders measures the cycle (stride) length as the distance between the first four footprints only and so did not include the distance to the return of the initial foot to the ground as is normally done. If we use the latter, more typical measurement of the cycle length, then the overtracks of the hipparionine fossil horses were 0.19 and 0.27 times the distance of the cycle length (as opposed to 0.27 and 0.31; Renders, 1984a, p. 180). This overtrack length is still considerable and illustrates that at very fast running walks, the overstepping can be so large as to form diagonal instead of lateral couplets (as is seen in Renders, 1984a, fig. 2).
The possibility that ancient horses could rack or tölt or pace (as well as display the running walk) is an interesting find in light of recent studies in horse genetics indicating that modern horses possess a so-called “gait-keeper gene” (DMRT3) that limits the gaits and speeds of which they are capable (Andersson et al., 2012; Kristjansson et al., 2014; Promerová et al., 2014; Jäderkvist Fegraeus et al., 2015, 2017; Regatieri et al., 2016). In gaited horses the DMRT3 gene has a mutation that results in a shorter and less functional protein unit. The normal DMRT3 gene yields a protein that is active in the spines of mammals and helps to coordinate their limb movements between front and hind and between the opposite sides of the body. The mutated form of the gene makes it harder for young animals to coordinate their hind limbs and transition to a gallop. Hence horse breeds with the mutation, such as Standardbred, Paso Fino, and Icelandic horses, can more easily learn to pace or engage in some other “artificial” gait. The mutated DMRT3 gene has even been tracked back to a presumable first appearance in ninth-century England (Wutke, 2016). As wild horses also possess the normal DMRT3 gene, the fact that earlier horses could rack or tölt suggests there may be more to the story genetically, and perhaps horses developed genes that limited their gait possibilities but allowed for the easier development of the three standard gaits of walk, trot, and gallop as this was beneficial to their survival. In any case, more recent studies have suggested a more complicated genetical picture for horse gaits as other genes than DMRT3 seem to be involved in such gaits as the fox trot and whether or not a horse has a tendency to trot or pace (Gonçalves Fonseca et al., 2017; Staiger et al., 2017; Novoa-Bravo et al., 2018; McCoy et al., 2019).
Other fossil horse hoof prints that have been examined, diagrammed, or photographed are not fully gaitable by my estimation. They often occur as single impressions or couplets, in which case reconstructing the exact gait is not possible. For example, there is an early Miocene (ca. 16.5 Ma) horse footprint couplet present in the Barstow Formation of Prosperity Canyon, western Calico Mountains, California (Reynolds, 2006, fig. 1). The hoof impressions are 2.95 cm in length with an overtrack roughly equal to the size of the hoof, or around 2.5 cm, with a slight lateral offset between the pair of impressions. This is hence a large overtrack and suggests a faster gait such as a trot, pace, or canter. However, lacking other foot impressions, it is not possible to eliminate any of these possibilities or even if it is a fast walk.
On other occasions there are multiple fossilized horse hoof prints, but the opposite problem of an overabundance of wealth is they occur as trample grounds wherein multiple individuals step near and on top of each other's tracks, making it hard to determine which impressions belong to the same individual. Such is the case with the late Hemingfordian (He 2) tracks of the Muddy Creek Formation in Moapa, Nevada, where footprints 3.2 cm long formed around 17 Ma (Reynolds, 2006, fig. 2). It is also the case with the Pliocene lacustrine “Barnyard” site at Copper Canyon in Death Valley, California, of early Blancan sediments (Bl 1) of around 4.25 Ma. Here likely Hipparion or Neohipparion set down multiple prints 9.2–10.8 cm long (Santucci et al., 2014, fig. 7; Nyborg et al., 2012, fig. 7f). Similar are the late Pliocene (Blancan 3) Dinohippus [?] hoof imprints measuring 6.2 cm that formed around 2 Ma in fluvial–alluvial sediments of the Ocotillo Formation, Vallecito–Fish Creek Basin, in Anza-Borrego Desert State Park, California (Remeika, 2006), and Pleistocene (late Irvingtonian, Ir 2) tracks 10–12.5 cm long formed around 1 Ma in Lake Tacoma sediments near Shoshone, Death Valley, California (Reynolds, 1999). As it stands, it is very hard to distinguish individual trackways from the available photographs and illustrations. More fieldwork in these areas may be able to isolate footprint pathways made by a single individual and hence allow the more exact determination of a horse gait.
In closing let it be noted that determining the gaits of fossil horses from their tracks can be difficult as trackways may be trampled upon by other animals, and so isolating individual sequences of prints can be quite challenging. In addition the preserved portion of trackways are usually minimal, and typically there are not enough footprints preserved to make determination of a gait possible. Finally, it may be difficult to distinguish front and hind footprints due to minimal differences between them in the horse species or due to the quality of the imprint on account of the substrate, and the exact species and size of the horse making the track may not be well known.
Still, as has long been noted, gaits involve unique patterns of limb coordination and footfalls, and these are often reflected in observable patterns in footprints made (see Fig. 1 below as well as Jordan, 1910, p. 274; Smith, 1912, p. 635; Renders, 1984a, b; Kienapfel et al., 2014). Moreover, by attending to overall stride length, distances between pairs of prints (intercouplet distance), distances of overtracking, and arrangements between closely impacting pairs of feet (lateral offset and cross-measure), determination of a horse's gait from footprint measurements is often possible.
Quite extraordinarily, in applying the above methodology in order to determine the gaits displayed by fossil horses (in conjunction with the studies of Renders, 1984a, b, and Kienapfel et al., 2014), it appears that Miocene to Pliocene horses did not just walk and presumably trot and gallop but could also engage in the so-called “artificial” gaits of the rack or tölt and running walk. All of this suggests that early horses were clearly “gaited” and capable of a wide range of gaits, perhaps helping them outmaneuver prey on different terrains and also effectively migrate from place to place. This wide range of gaits available to earlier horses seems to have been reduced in modern breeds through selection and breeding, thus reducing the pool of available gaits to the four natural gaits (walk, trot, canter, gallop) found in most horse breeds.
At any rate it is hoped that this study, in combination with those of others, will help resolve gaits exhibited by fossilized horse prints in the future. Further work would be beneficial on living horses vis-à-vis measuring the exact speed of the gait via videos or speed guns and correlating this with gait variations, including detailed measurements of interior straddle and degree of hoof rotation away from the centerline or nearest impression as well as how many parallel tracks of prints occur in any particular gait and their arrangement. Much more work on the kinematics and characteristics of the slow gait, rack, pace, and fox trot in the moving horse and associated footprints needs to be done at slow and fast speeds. In addition studies of hoofprints made by unshod horses would be desirable as these would mimic the natural state of extinct horse species. Finally the study of Kienapfel et al. (2014), in conjunction with my calculations for estimated speed using the formula in Alexander (1976), suggests that Alexander's formula works well for faster horse gaits but overestimates the speed of slower horse gaits, and this is a line of research that could be pursued. Such an investigation could also look at the relation between overall body proportions such as the ratio of the limb length trunk length and how this affects gait characteristics in horses, camels, tapirs, and rhinos at different ages.
The Scaphohippus intermontanus footprints and molds are housed at the San Bernardino County Museum, Redlands, California: location SBCM 1-130-394, holotype SBCM L1816-3436. There is also a third metatarsal of Scaphohippus intermontanus around 10.7 cm long housed at the San Bernardino County Museum (L1585-56) but from considerably older Davis Ranch strata, Cajon Valley Formation, Unit 3 (ca. 16–17 Ma). The Scaphohippus intermontanus skull is housed at the American Museum of Natural History in New York (AMNH: FM 87301). The Equus conversidens footprints are housed at the Royal Alberta Museum, Edmonton, Alberta: specimen DhPg-8 3840-3843. The Eurygnathohippus hasumense footprints are still located at Site G in Laetoli, Tanzania.
The supplement related to this article is available online at: https://doi.org/10.5194/fr-24-151-2021-supplement.
The author declares that there is no conflict of interest.
The author wishes to thank Margaret Resketo, Anne Gunther, Derk Adams, Jennifer Gardener, Jessica Farrell, Victoria Chaidez, Aretha Crout, Nani Barnes, Flora Jean Weiss, Cynthia Binder, and Kimberly Anderson, who aided in studies on the footfall patterns of living horses as equestrians or research assistants.
This paper was edited by Rene Hoffmann and reviewed by Holger Preuschoft and one anonymous referee.
Alberdi, M. T., Prado, J. L., and Ortiz-Jaureguizar, E.: Patterns of body size changes in fossil and living equini (Perissodacytla), Biol. J. Linn. Soc., 54, 349–370, https://doi.org/10.1016/0024-4066(95)90015-2, 1995.
Alexander, R. M.: Estimates of speeds of dinosaurs, Nature, 261, 129–130, https://doi.org/10.1038/261129a0, 1976.
Alf, R. M.: Mammal trackways from the Barstow Formation, Bull. S. Calif. Acad. Sci., 65, 258–264, 1966.
Andersson, L. S., Lahrhammar, M., Memic, F., Wootz, H., Schwochow, D., Rubin, C.-J., Patra, K., Arnason, T., Wellbring, L., Hjälm, G., Imsland, F., Petersen, J. L., McCue, M. E., Mickelson, J. R., Cothran, G., Ahituv, N., Roepstorff, L., Mikko, S., Vallstedt, A., Lindgren, G., Andersson, L., and Kullander, K.: Mutations in DMRT3 affect locomotion in horses and spinal circuit function in mice, Nature, 488, 642–646, https://doi.org/10.1038/nature11399, 2012.
Armour-Chelu, M. and Bernor, R. L.: Equidae, in: Paleontology and Geology of Laetoli: Human Evolution in Context: Volume 2: Fossil Hominins and the Associated Fauna, edited by: Harrison, T., Springer, 295–326, Dordrecht, the Netherlands, 2011.
Back, W., Schamhardt, H. C., and Barneveld, A.: Kinematic comparison of the leading and trailing fore- and hindlimbs at the canter, Equine Vet. J. Sup., 23, 80–83, https://doi.org/10.1111/j.2042-3306.1997.tb05060.x, 1997.
Barrey, E.: Methods, applications and limitations of gait analysis in horses, Vet. J., 157, 7–22, https://doi.org/10.1053/tvjl.1998.0297, 1999.
Barrey, E.: Gaits and interlimb coordination, in: Equine locomotion, edited by: Beck, W. and Clayton, H. M., Saunders, 85–97, Philadelphia, Pennsylvania, 2013b.
Bernor, R. L., Haile-Selasse, Y., and Scott, R. S.: A contribution to the evolutionary history of Ethiopian hipparionine horses (Mammalia, Equidae): Morphometric evidence from the postcranial skeleton, Geodiversitas, 27, 133–158, 2005.
Bernor, R. L., Armour-Chelu, M., Gilbert, W., Kaiser, T. M., and Schulz-Kornas, E.: Equidae, in: Cenozoic Mammals of Africa, University of California Press, 685–721, 2010.
Bertram, J. E. A. and Gutmann, A.: Motions of the running horse and cheetah revisited: Fundamental mechanics of the transverse and rotary gallop, Interface, 6, 549–559, https://doi.org/10.1098/rsif.2008.0328, 2009.
Biancardi, C. M. and Minetti, A. E.: Biomechanical determinants of transverse and rotary gallop in cursorial mammals, J. Exp. Biol., 215, 4144–4156, https://doi.org/10.1242/jeb.073031, 2012.
Boehart, S., Marquis, H., Falaturi, P., and Carstanjen, B.: Influence of palmarly added weights on locomotor parameters of the tölt of Icelandic Horse and comparison with corresponding data of the flying pace, Pferdeheilkunde, 29, 628–632, 2013.
Bordy, E. M., Rampersadh, A., Abrahams, M., Lockley, M. G., and Head, H. V: Tracking the Pliensbachian–Toarcian Karoo firewalkers: Trackways of quadruped and biped dinosaurs and mammaliaforms, PLoS One, 15, e0226847, https://doi.org/10.1371/journal.pone.0226847, 2020.
Cabral-Perdomo, M. A., Bravo-Cuevas, V. M., Perez-Perez, A., and Garcia-Cabrera, N.: Descripción de las huellas de camélidos y félidos de la localidad Pie de Vaca, Cenozoico Tardío de Puebla, centro de México y algunas consideraciones paleobiológicas, B. Soc. Geol. Mex., 70, 397–416, https://doi.org/10.18268/bsgm2018v70n2a9, 2018.
Chrószcz, A., Janeczek, M., Pasicka, E., and Klećkowska-Nawrot, J.: Height at the withers estimation in the horses based on the internal dimension of cranial cavity, Folia Morphol., 73, 143–148, https://doi.org/10.5603/FM.2014.0021, 2014.
Clayton, H. M.: The extended canter: A comparison of some kinematic variables in horses trained for dressage and for racing, Acta Anat., 146, 183–187, https://doi.org/10.1159/000147443, 1993.
Clayton, H. M.: Comparison of the stride kinematics of the collected, working, medium and extended trot in horses, Equine Vet. J., 26, 230–234, https://doi.org/10.1111/j.2042-3306.1994.tb04375.x, 1994.
Clayton, H. M.: Comparison of the stride kinematics of the collected, medium, and extended walks in horses, Am. J. Vet. Res., 56, 849–852, 1995.
Clayton, H. M. and Bradbury, J. W.: Temporal characteristics of the fox trot, a symmetrical equine gait, Appl. Anim. Behav. Sci., 42, 153–159, https://doi.org/10.1016/0168-1591(94)00539-Q, 1995.
Clayton, H. M. and Hobbs, S. J.: Review of biomechanical gait classification with reference to collected trot, passage and piaffe in dressage horses, Animals, 9, 763, https://doi.org/10.3390/ani9100763, 2019.
Deuel, N. R. and Lawrence, L. M.: Gallop velocity and limb contact variables of quarter horses, J. Equine Vet. Sci., 6, 143–147, https://doi.org/10.1016/S0737-0806(86)80064-8, 1986.
Deuel, N. R. and Park, J.-J.: Canter lead change kinematics of superior Olympic dressage horses, J. Equine Vet. Sci., 10, 287–298, https://doi.org/10.1016/S0737-0806(06)80013-4, 1990.
Drevemo, S., Dalin, G., Fredricson, I., and Hjertén, G.: Equine locomotion: 1. The analysis of linear and temporal stride characteristics of trotting Standardbreds, Equine Vet. J., 12, 60–65, https://doi.org/10.1111/j.2042-3306.1980.tb02310.x, 1980a.
Drevemo, S., Fredricson, I., Dalin G., and Björne, K.: Equine locomotion: 2. The analysis of coordination between limbs of trotting Standardbreds, Equine Vet. J., 12, 66–70, https://doi.org/10.1111/j.2042-3306.1980.tb02311.x, 1980b.
Feranec, R. S. and Pagnac, D. C.: Hypsodonty, horses, and the spread of C4 grasses during the middle Miocene in Southern California, Evol. Ecol. Res., 18, 201–223, 2017.
Gonçalves Fonseca, M., De Camargo Ferraz, G., Lage, J., Luis Pereira, G., and Abdallah Curic, R.: A genome-wide association study reveals differences in the genetic mechanism of control of the two gait patterns of the Brazilian Mangalarga Marchador Breed, J. Equine Vet. Sci., 53, 64–67, https://doi.org/10.1016/j.jevs.2016.01.015, 2017.
Gonçalves Fonseca, M., Silvatti, A., and Lage, J.: Kinematics of marcha picada of Mangalarga Marchador horses with AA and AC genotypes of DMRT3, Comp. Exerc. Physiol., 14, S51, 2018.
Gray, J.: Animal locomotion, Weidenfield and Nicolson, London, 265–281, 1968.
Grogan, J. W.: The gaits of horses, J. Am. Vet. Med. Assoc., 118, 112–117, 1951.
Gunnarsson, V., Stefánsdóttir, G. J., Jansson, A., and Roepstorff, L.: The effect of rider weight and additional weight in Icelandic horses in tölt: Part II, Stride parameters responses, Animal, 11, 1567–1572, https://doi.org/10.1017/S1751731117000568, 2017.
Harris, A. H. and Porter, L. S. W.: Late Pleistocene Horses of Dry Cave, Eddy County, New Mexico, J. Mammal., 61, 46–65, https://doi.org/10.2307/1379956, 1980.
Harris, S. E.: Horse gaits, balance and movement, Turner, Nashville, Tennessee, 2016.
Henderson, D. M.: Burly gaits: Centers of mass, stability, and the trackways of sauropod dinosaurs, J. Vertebr. Paleontol., 26, 907–921, https://doi.org/10.1671/0272-4634(2006)26[907:BGCOMS]2.0.CO;2, 2006.
Hildebrand, M.: Symmetrical gaits of horses, Science, 150, 701–708, https://doi.org/10.1126/science.150.3697.701, 1965.
Hildebrand, M.: Analysis of asymmetrical gaits, J. Mammal., 58, 131–156, https://doi.org/10.2307/1379571, 1977.
Hildebrand, M.: The adaptive significance of tetrapod gait selection, Am. Zool., 20, 255–267, 1980.
Hobbs, S. J., Bertram, J. E., and Clayton, H. M.: An exploration of the influence of diagonal dissociation and moderate changes in speed on locomotor parameters in trotting horses, PeerJ, 4, e2190, https://doi.org/10.7717/peerj.2190, 2016.
Holmström, M., Fredricson, I., and Drevemo, S.: Biokinematic analysis of the Swedish Warmblood riding horse at trot, Equine Vet. J., 26, 235–240, https://doi.org/10.1111/j.2042-3306.1994.tb04376.x, 1994.
Hooijer, D. A.: Miocene to Pleistocene Hipparions of Kenya, Tanzania, and Ethiopia, Zool. Verh., 142, 3–80, 1975.
Hooijer, D. A.: Hipparions of the Laetoli beds, Tanzania, in: Laetoli: A Pliocene Site in Northern Tanzania, edited by: Leakey, M. D. and Harris, J. M., Clarendon Press, 301–312, 1987.
Jäderkvist Fegraeus, K., Freyja Imsland, N. H, Árnason, T., Andersson, L., Andersson, L. S., and Lindgrena, G.: The importance of the DMRT3 “Gait keeper” mutation on riding traits and gaits in Standardbred and Icelandic horses, Livest. Sci., 176, 33–39, https://doi.org/10.1016/j.livsci.2015.03.025, 2015.
Jäderkvist Fegraeus, K., Hirschberg, I., Árnason, Andersson, L., Velie, B. D., Andersson, L. S., and Lindgren, G.: To pace or not to pace: a pilot study of four- and five-gaited Icelandic horses homozygous for the DMRT3 “Gait Keeper” mutation, Anim. Genet., 48, 694–697, https://doi.org/10.1111/age.12610, 2017.
Jordan, R.: The gait of the American trotter and pacer, William R. Jenkins, New York, 1910.
Kienapfel, K., Läbe, S., and Preuschoft, H.: Do tracks yield reliable information on gaits? – Part 1: The case of horses, Foss. Rec., 17, 59–67, https://doi.org/10.5194/fr-17-59-2014, 2014.
Kristjansson, T., Bjornsdottir, S., Sigurdsson, A., Andersson, L. S., Lindgren, G., Helyar, S. J., Klonowski, A. M., and Arnason, T.: The effect of the “gait keeper” mutation in the DMRT3 gene on gaiting ability in Icelandic horses, J. Anim. Breed. Genet., 131, 415–425, https://doi.org/10.1111/jbg.12112, 2014.
Leach, D. H. and Dagg, A. I.: A review of research on equine locomotion and biomechanics, Equine Vet. J., 15, 93–102, https://doi.org/10.1111/j.2042-3306.1983.tb01726.x, 1983.
Leach, D. H., Ormrod, K., and Clayton, H.: Standardised terminology for description and analysis of equine locomotion, Equine Vet. J., 16, 522–528, https://doi.org/10.1111/j.2042-3306.1984.tb02007.x, 1984.
Leach, D. H., Sprigings, E. J., and Laverty, W. H.: Multivariate statistical analysis of stride-timing measurements of nonfatigued racing Thoroughbreds, Am. J. Vet. Res., 48, 880–888, 1987.
Leleu, C., Cotrel, C., and Barrey, E.: Effect of age on locomotion of Standardbred trotters in training, Equine Comp. Exerc. Physiol., 1, 107–117, https://doi.org/10.1079/ECEP200312, 2004.
Loitsch, C.: Kinematische Untersuchung über den Canter von Pferden (Equus caballus), Ruhr Universität Bochum, 1993.
Morrice-West, A. V., Hitchens, P. L., Walmsley, E. A., Stevenson, M. A., Wong, A. S. M., and Whitton, R. C.: Variation in GPS and accelerometer recorded velocity and stride parameters of galloping Thoroughbred horses, Equine Vet. J., https://doi.org/10.1111/evj.13370, online first, 2020.
Mazzetta, G. V. and Blanco, E. R.: Speeds of dinosaurs from the Albian-Cenomanian of Patagonia and sauropod stance and gait, Acta Palaeontol. Pol., 46, 235–246, 2001.
McCoy, A. M., Beeson, S. K., Rubin, C.-J., Andersson, L., Caputo, P., Lykkjen, Moore, A., Piercy, R. J., Mickelson, J. R., and McCue, M. E.: Identification and validation of genetic variants predictive of gait in Standardbred horses, PLoS Genet., 15, e1008146, https://doi.org/10.1371/journal.pgen.1008146, 2019.
McNeil, P., Mills, L. V., Tolman, M. S., and Kooyman, B.: Significance of latest Pleistocene tracks, trackways, and trample grounds, from Southern Alberta, Canada, in: Cenozoic Vertebrate Tracks and Traces, edited by: Lucas, S. G., Spielmann, J. A., and Lockley, M. G., New Mexico Museum of Natural History and Science, 209–223, 2007.
Merriam, J. C.: Tertiary mammalian faunas of the Mojave Desert, Bull. Dept. Geol. Univ. Calif., 11, 437–585, 1919.
Nicodemus, M. C. and Booker, J. E.: Two-dimensional kinematics of the jog and lope of the stock breed western pleasure horse, Equ. Comp. Exer. Physiol., 4, 59–70, https://doi.org/10.1017/S1478061507811467, 2007.
Nicodemus, M. C. and Clayton, H. M.: Temporal variables of the 4-beat stepping jog and lope, in: Proceedings of the Seventeenth Equine Nutrition and Physiology Symposium, Equine Nutrition and Physiology Society, 247–252, 2001.
Nicodemus, M. C. and Clayton, H. M.: Temporal variables of four-beat, stepping gaits of gaited horses, Appl. Anim. Behav. Sci., 80, 133–142, https://doi.org/10.1016/S0168-1591(02)00219-8, 2003.
Nicodemus, M. C. and Slater, K.: Forelimb kinematics of the flat walk and fox trot of the Missouri Fox Trotter, Comp. Exerc. Physiol., 6, 149–156, https://doi.org/10.1017/S1755254010000048, 2009.
Nicodemus, M. C., Holt, K. M., and Swartz, K.: Relationship between velocity and temporal variables of the flat shod running walk, Eqine Veter. J. Sup., 34, 340–343, https://doi.org/10.1111/j.2042-3306.2002.tb05444.x, 2002.
Novoa-Bravo, M., Jäderkvist Fegraeus, K., Rhodin, M., Strand, E., García, L. F., and Lindgren, G.: Selection on the Colombian Paso Horse's gaits has produced kinematic differences partly explained by the DMRT3 gene, PLoS Genet., 14, e0212149, https://doi.org/10.1371/journal.pone.0202584, 2018.
Nyborg, T., Buccheim, P., and Nick, K. E.: Age, stratigraphy, depositional environment, and vertebrate ichnology of the Pliocene Copper Canyon Formation, Death Valley, California, in: Searching for the Pliocene: Southern Exposures, edited by: Reynolds, R. E., California State University Desert Studies Center, 114–124, 2012.
Onar, V., Kahvecioğlu, K. O., Olğun Erdikmen, D., Alpak, H., and Parkan Yaramiş, Ç: The estimation of withers height of ancient horse: New estimation formulations by using the metacarpal measurements of living horse, Rev. Med. Vet., 169, 157–165, 2018.
Pagnac, D.: Scaphohippus, a new genus of horse (Mammalia: Equidae) from the Barstow Formation of California, J. Mamm. Evol., 13, 37–61, https://doi.org/10.1007/s10914-005-9002-2, 2006.
Pajak, A. F. and Vincelette, A. R.: “Merychippus” stylodontus and “M”. intermontanus: Biostratigraphy and basic morphology of two Barstovian tridactyl horses, San Bernadino County Museum Assoc. Q., 38, 80–85, 1991.
Parsons, K. J., Pfau, T., and Wilson, A. M.: High-speed gallop locomotion in the Thoroughbred racehorse. I. The effect of incline on stride parameters, J. Exp. Biol., 211, 935–944, https://doi.org/10.1242/jeb.006650, 2008.
Pecha, A., Rumpler, B., Kotschwar, A., and Licka, T.: Der Einfluss von unterschiedlich schweren Ballenboots in zwei verschiedenen Tempi auf die Dauer und den Beginn der Stützbeinphase in der Gangart Tölt des Islandpferdes, Pferdeheilkunde 27, 687–694, 2011.
Promerová, M., Andersson, L. S., Juras, R., Penedo, M. C. T., Reissmann, M., Tozaki, T., Bellone, R., Dunner, S., Hořín, P., Imsland, F., Imsland, P., Mikko, S., Modrý, D., Roed, K. H., Schwochow, D., Vega-Pla, J. L., Mehrabani-Yeganeh, H., Yousefi-Mashouf, N., Cothran, E. G., Lindgren, G., and Anderssonet, L.: Worldwide frequency distribution of the “Gait keeper” mutation in the DMRT3 gene, Anim. Genet., 45, 274–282, https://doi.org/10.1111/age.12120, 2014.
Prost, J. H.: Gaits of monkeys and horses: A methodological critique, Am. J. Physiol. Anthropol., 32, 121–128, https://doi.org/10.1002/ajpa.1330320114, 1970.
Regatieri, I. C., Eberth, J. E., Sarver, F., Lear, T. L., and Bailey, E.: Comparison of DMRT3 genotypes among American Saddlebred horses with reference to gait, Anim. Genet., 47, 603–605, https://doi.org/10.1111/age.12458, 2016.
Remeika, P.: Fossil footprints of Anza-Borrego, in: Fossil Treasures of the Anza-Borrego Desert: The Last Seven Million Years: El Cajon, edited by: Jefferson, G. T. and Lindsay, L., Sunbelt Publications, San Diego, California, 311–327, 2006.
Renders, E.: The gait of Hipparion sp. from fossil footprints in Laetoli, Tanzania, Nature, 308, 179–181, https://doi.org/10.1038/308179a0, 1984a.
Renders, E.: Corrigendum to “The gait of Hipparion sp. from fossil footprints in Laetoli, Tanzania”, Nature, 308, 866, https://doi.org/10.1038/308866a0, 1984b.
Reynolds, R. E.: Pleistocene mammal tracks near Shoshone, southern Death Valley, California, San Bernadino County Museum Assoc. Q., 46, 27–29, 1999.
Reynolds, R. E.: Horse hoof prints in the fossil record, in: Making Tracks Across the Southwest: The 2006 Desert Symposium, edited by: Reynolds, R. E., California State University, Desert Studies Consortium and LSA Associates, 25–28, 2006.
Robilliard, J. J., Pfau, T., and Wilson, A. M.: Gait characterisation and classification in horses, J. Exp. Biol., 210, 187–197, https://doi.org/10.1242/jeb.02611, 2007.
Sarjeant, W. A. S. and Reynolds, R. E.: Camel and horse footprints from the Miocene of California and Nevada, San Bernadino Museum Assoc. Q., 46, 3–20, 1999.
Santucci, V. L., Tweet, J., Bustos, D., Nyborg, T., and Hunts, A. P.: An inventory of Cenozoic Fossil Vertebrate Tracks and Burrows in National Park Service Areas, New Mexico Museum Nat. Hist. Sci. Bull., 62, 469–488, 2014.
Seder, J. A. and Vickery, C. E.: Double and triple fully airborne phases in the gaits of racing speed Thoroughbreds, J. Equine Vet. Sci., 23, S73–S81, https://doi.org/10.1016/S0737-0806(03)70018-5, 2003a.
Seder, J. A. and Vickery, C. E.: Temporal and kinematic gait variables of Thoroughbred racehorses at or near racing speeds, J. Equine Vet. Sci., 23, S82–S112, https://doi.org/10.1016/S0737-0806(03)70019-7, 2003b.
Serra Bragança, F. M., Broomé, S., Rhodin, M., Björnsdóttir, S., Gunnarsson, V., Voskamp, J. P., Persson-Sjodin, E., Back, W., Lindgren, G., Novoa-Bravo, M., Roepstorff, C., Van der Zwaag, B. J., Van Weeren, P. R., and Hernlund, E.: Improving gait classification in horses by using inertial measurement unit (IMU) generated data and machine learning, Sci. Rep., 10, 17785, https://doi.org/10.5167/uzh-192795, 2020.
Serra Bragança, F. M., Broomé, S., Rhodin, M., Björnsdóttir, S., Gunnarsson, V., Voskamp, J. P., Persson-Sjodin, E., Back, W., Lindgren, G., Novoa-Bravo, M., Roepstorff, C., Van der Zwaag, B. J., Van Weeren, P. R., and Hernlund, E.: Corrigendum to “Improving gait classification in horses by using inertial measurement unit (IMU) generated data and machine learning”, Sci. Rep., 11, 9379, https://doi.org/10.1038/s41598-020-73215-9, 2021.
Smith, F.: A manual of veterinary physiology, Baillière, Tindall, and Cox, London, 1912.
Solé, M., Gómez, M. D., Martínez Galisteo, A., Santos, R., and Valera, M.: Kinematic characterization of the Menorca Horse at the walk and the trot: Influence of hind limb pastern angle, J. Equine Vet. Sci., 33, 726–733, https://doi.org/10.1016/j.jevs.2012.12.002, 2013.
Splan, R. K. and Hunter, H. B.: Temporal variables of the canter of the Tennessee Walking Horse, Equine Comp. Exerc. Physiol., 1, 41–44, https://doi.org/10.1079/ECP20033, 2004.
Stachurska, A., Kolstrung, R., Pięta, M., and Silmanowicz, P.: Differentiation between fore and hind hoof dimensions in the horse (Equus caballus), Arch. Tierzucht, 51, 531–540, https://doi.org/10.5194/aab-51-531-2008, 2008.
Stachurska, A., Kolstrung, R., Pięta, M., and Silmanowicz, P.: Hoof size as related to body size in the horse (Equus caballus), Anim. Sci. Pap. Rep., 29, 213–222, 2011.
Staiger, E. A., Almén, M. S., Promerová, M., Brooks, S., Cothran, E. G., Imsland, F., Jäderkvist Fegraeus, K., Lindgren, G., Mehrabani Yeganeh, H., Mikko, S., Vega-Pla, J. L., Tozaki, T., Rubin, C. J., and Andersson, L.: The evolutionary history of the DMRT3 “Gait keeper” haplotype, Anim. Genet., 48, 551–559, https://doi.org/10.1111/age.12580, 2017.
Starke, S. D., Robilliard, J. J., Weller, R., Wilson, A. M., and Pfau, T.: Walk-run classification of symmetrical gaits in the horse: A multidimensional approach, J. Roy. Soc. Interface, 6, 335–342, https://doi.org/10.1098/rsif.2008.0238, 2009.
Stefánsdóttir, G. J., Jansson, A., Ragnarsson, S., and Gunnarsson, V.: Speed of gaits in Icelandic horses and relationships to sex, age, conformation measurements and subjective judges' scores, Comp. Exerc. Physiol., 17, 151–160, https://doi.org/10.3920/CEP200039, 2021.
Stevens, K. A., Ernst, S., and Marty, D.: Uncertainty and ambiguity in the interpretation of sauropod trackways, in: Dinosaur Tracks: The Next Steps, edited by: Falkingham, P. L., Marty, D., and Richter, A., Indiana University Press, 227–244, 2016.
Streitlein, I. and Preuschoft, H.: Die Kinematik der Trabtempi von Reitpferden, in: Studien zu den Bewegungen von Sportpferden, edited by: Preuschoft, H., Fritz, M., Huellen-Kluge, K., Knisel, G., and Streitlein, I., Deutsche Reiterliche Vereinigung, Warendorf, Germany, 20–65, 1987.
Takahashi T., Aoki, O., and Hiraga, A.: Running form characteristics of the Triple Crown winner in Japan, J. Equine Vet. Sci., 18, 47–53, https://doi.org/10.1294/jes.18.47, 2007.
Thompson, M. E., White Jr., R. S., and Morgan, G. S.: Pace versus trot: Can medium speed gait be determined from fossil trackways?, in: Cenozoic Vertebrate Tracks and Traces, edited by: Lucas, S. G., Spielmann, J. A., and Lockley, M. G., New Mexico Museum of Natural History and Science, 309–314, 2007.
Thulborn, R. A.: Speeds and gaits of dinosaurs, Palaeogeogr. Palaeocl., 38, 227–256, https://doi.org/10.1016/0031-0182(82)90005-0, 1982.
Thulborn, R. A.: The gait of dinosaurs, in: Dinosaur Tracks and Traces, edited by: Gillett, D. D. and Lockley, M. G., Cambridge University Press, 39–50, 1989.
Van der Made, J.: The large mammals of the Plio-Pleistocene of Africa: Afrotheria, Perissodactyla and Artiodactyla I, in: La Cuna de la Humanidad, edited by: Enríquez, S. and Alvarado, E., Museo Arquelógico Regional, Alcalá de Henares & Museo de la Evolución Humana, 324–336, 2014.
Waldern, N. M. Wiestner, T., Ramseier, L. C., and Weishaupt, M. A.: Comparison of limb loading and movement of Icelandic horses while tölting and trotting at equal speeds, Am. J. Vet. Res., 76, 1031–1040, https://doi.org/10.2460/ajvr.76.12.1031, 2015.
Walker, V. A., Tranquille, C. A., Newton, J. R., Dyson, S. J., Brandham, J., Northrop, A. J., and Murray, R. C.: Comparison of limb kinematics between collected and lengthened (medium/extended) trot in two groups of dressage horses on two different surfaces, Equine Vet. J., 49, 673–680, https://doi.org/10.1111/evj.12661, 2017.
Webb, S. D.: Locomotor evolution in camels, Forma Functio, 5, 99–112, 1972.
Weishaupt, M. A., Byström, A., Geser-von Peinen, K., Wiestner, T., Meyers, H., Waldern, N., Johnston, C., Van Weeren, R., and Roepstorff, L.: Kinetics and kinematics of the passage, Equine Vet. J., 41, 263–276, https://doi.org/10.2746/042516409x397226, 2009.
Weishaupt, M. A., Hogg, H. P., Auer, J. A., and Weistner, T.: Velocity-dependent changes of time, force and spatial parameters in Warmblood horses walking and trotting on a treadmill, Equine Vet. J. Sup., 38, 530–537, https://doi.org/10.1111/j.2042-3306.2010.00190.x, 2010.
Wilson, B. D., Neal, R., Howard, A., and Groenendyk, S.: The gait of pacers 1: Kinematics of the racing stride, Equine Vet. J., 20, 341–346, https://doi.org/10.1111/j.2042-3306.1988.tb01542.x, 1988a.
Witte, T. H., Hirst, C. V., and Wilson, A. M.: Effect of speed on stride parameters in racehorses at gallop in field conditions, J. Exp. Biol., 209, 4389–4397, https://doi.org/10.1242/jeb.02518, 2006.
Wutke, S.: The origin of ambling horses, Curr. Biol., 26, R697–R699, https://doi.org/10.1016/j.cub.2016.07.001, 2016.
Ziegler, L.: Easy-gaited horses, Storey Publishing, North Adams, Massachusetts, 2005.
Zips, S., Peham, C., Scheidl, M., Licka, T., and Girtler, D.: Motion pattern of the toelt of Icelandic horses at different speeds, Equine Vet. J., 33, 109–111, https://doi.org/10.1111/j.2042-3306.2001.tb05371.x, 2001.
This species, which was formerly placed in the genus Merychippus, albeit with some hesitancy, by Pajak and Vincelette (1991), has been reassigned more recently to Scaphohippus intermontanus (Pagnac, 2006).
Some of the African hipparionine horses, including the species studied here, have, of late, been reassigned to Eurygnathohippus hasumense (Hooijer, 1987; Armour-Chelu and Bernor, 2011; Bernor et al., 2005, 2010; Van der Made, 2014).