the Creative Commons Attribution 4.0 License.
the Creative Commons Attribution 4.0 License.
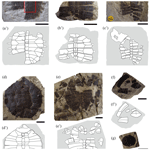
Ontogenetic development of the European basal aquatic turtle Pleurosternon bullockii (Paracryptodira, Pleurosternidae)
Andrea Guerrero
Adán Pérez-García
Several British specimens of relatively complete and partial shells of small pleurosternid turtles, found in the Purbeck Limestone Group (Berriasian, Lower Cretaceous), are analysed in detail here. Despite having been found more than a century ago, most of them remained unpublished until now. Due to the scarce knowledge available to date about the small individuals of Pleurosternidae from the British record, their taxonomic status was doubtful. Thus, some authors proposed their attribution to a new but not defined taxon of small size, whereas others suggested that they could represent juvenile individuals of Pleurosternon bullockii, the adults of which were also found in the Purbeck Limestone Group but at different levels. Knowledge about the shell of the adult individuals of Pleurosternon bullockii has notably increased recently, abundant information on its intraspecific variability being currently available. In this context, a detailed study of the small specimens through qualitative and quantitative approaches is performed here. The results are evidence a significant range of shape variability because of ontogenetic development, as well as other types of intraspecific variation such as inter-individual variation. As a consequence, their attribution to juvenile individuals of Pleurosternon bullockii is justified, providing new data on the ontogenetic development of a basal form.
- Article
(14907 KB) -
Supplement
(1469 KB) - BibTeX
- EndNote
The European uppermost Jurassic to lowermost Cretaceous (Tithonian to Berriasian) Pleurosternon bullockii (Owen, 1853) corresponds to the best-represented member of Pleurosternidae (Guerrero and Pérez-García, 2021), a relatively diverse group of Upper Jurassic–Lower Cretaceous North American and European freshwater to brackish turtles (Gaffney, 1979; Pérez-García and Ortega, 2011; Pérez-García, 2014; Pérez-García et al., 2014, 2015). This lineage of Paracryptodira, together with its exclusively North American sister group Baenidae, constitutes the clade Baenoidea (Joyce et al., 2020; Pérez-García, 2020a). Paracryptodira is identified as a lineage of basal turtles (stem Testudines) closely related to the crown group (Pérez-García, 2020b).
The British turtle Pleurosternon bullockii was the first described pleurosternid. Most of the specimens currently known were found more than a century ago, in several localities of southern Great Britain, in Berriasian (Lower Cretaceous) levels of the Purbeck Limestone Group (Milner, 2004). The British collection of P. bullockii had not been analysed and figured in detail until recently (Guerrero and Pérez-García, 2020, 2021). In these last studies, a detailed approach to the knowledge about the inter-individual variation (i.e. polymorphisms and sexual dimorphism) for the adult stage of P. bullockii was obtained, an emended diagnosis for this species having been proposed. However, despite this noticeable advance in knowledge about the adult individuals of this species, the systematic determination of several small individuals of Pleurosternidae found in the same area remains uncertain. Thus, they show a controversial taxonomic status due to some authors proposing their attribution to a putative and undefined new genus of small size (Lapparent de Broin and Murelaga, 1999), whereas others suggested that they probably are juvenile individuals of P. bullockii (Lydekker, 1889a; Milner, 2004).
In this context, a detailed study of the small specimens of Pleurosternidae found in the British Purbeck Limestone Group has been carried out here through both qualitative and quantitative approaches. Most of them are unpublished specimens, only some individuals being previously figured in the literature but so far not analysed in detail. Thus, the aim of this study is to evaluate if these small forms belong to a new small taxon or justify their attribution to P. bullockii and, therefore, to provide data on the ontogenetic development of this basal form.
Institutional abbreviations
CAMSM, Sedgwick Museum, Department of Geology, University of Cambridge, UK; DORCM, Dorset County Museum, Dorchester, UK; NHMUK (formerly BMNH), Natural History Museum, London, UK; YPM, Peabody Museum of Natural History, Yale University, USA.
A total of 15 juvenile specimens recognized here, in a preliminary way, as potentially attributable to the species Pleurosternon bullockii are analysed in detail. They are partial or relatively complete shells (Figs. 1–4). In addition, some isolated plates are also recognized (Figs. 2d, 3c, 4e). Most of these individuals were never published, only one of the two slabs of NHMUK 48262 (Fig. 1a) having been previously figured through both drawings (Lydekker, 1889a, fig. 3) and photographs (Milner, 2004, fig. 6). In fact, the same slice was the only remain of a juvenile individual of the taxon considered in the preliminary descriptions (see Lydekker, 1889a, b). Despite remaining unpublished until now, most of the specimens studied here (i.e. NHMUK 48252; NHMUK 48263; NHMUK 48263a; NHMUK 48263c, NHMUK 48263e; NHMUK 48343; NHMUK 48344; NHMUK 48346; NHMUK 48347; NHMUK 48351; NHMUK 48353) were listed by previous authors (e.g. Lydekker, 1889b; Milner, 2004), some characters of the shell of some of them having been referred to (i.e. NHMUK 48343; NHMUK 48347; Lapparent de Broin and Murelaga, 1999).
Both qualitative and quantitative approaches have been performed to analyse the morphologic variation recognized as being related to the ontogenetic development in the taxon studied here. For the qualitative analysis, a detailed description of the shell elements (both plates and scutes) of the small individuals was carried out, as well as a comparison with the mature stage of P. bullockii based on the detailed information of the morphological variability in the shell from our recently published paper (Guerrero and Pérez-García, 2021). Photographs and schematic drawings of each of the specimens are included here (Figs. 1–4). The characters used by previous authors, in a preliminary way, to support the possible attribution of some of these specimens to juvenile individuals of P. bullockii (see Lydekker, 1889a, b) or to a new but not defined form (Lapparent de Broin and Murelaga, 1999) have been evaluated. In addition, other elements considered as ontogenetically variable but that have not been previously considered for the juveniles of P. bullockii have been analysed. These specimens have been compared with the characters of the shell that are ontogenetically variable in juveniles of other extinct (e.g. Brinkman et al., 2013; Jansen and Klein, 2014; Szczygielski et al., 2018; Li et al., 2019) and extant turtles (e.g. Somers et al., 2000; Pritchard, 2008; Fish and Stayton, 2014; Pate and Salmon, 2017). The initial distinction among the different ontogenetic stages, “juvenile”, “subadult” and “adult”, was based on both the size (see Sect. S1 of the Supplement) and the morphology of the shell elements (see Sect. 3).
Two-dimensional geometric morphometric method was performed for the most variable and best-preserved plates (i.e. nuchal and entoplastron), scutes (i.e. third vertebral) and regions of the shell (anterior plastral lobe) (see Sect. S2 for the sample size used in each analysis). All specimens were photographed using a relatively long camera–subject distance to minimize the parallax effect (Mullin and Taylor 2002). The tpsUtil v. 1.76 programme (Rohlf, 2012, 2015) was used to compile and convert the image files analysed here.
The two-dimensional geometric morphometric protocol described by Zelditch et al. (2012) was adopted. The selection of landmarks (see Sects. S3 to S6 for the set of landmarks used for each element) was based on their ability to represent the geometric forms (Larson, 2002; Murta-Fonseca and Fernandes, 2016). In addition, semi-landmarks were added for the analyses of the anterior plastral lobe and the entoplastron (see Sects. S5 and S6 for more details) in order to capture the shape along their curves (Gunz and Mitteroecker, 2013). The straight edges were used to scale each digitized individual. Landmarks and semi-landmarks were digitized using the tpsDig v.2.16 software (Rohlf, 2010, 2015). Following the same methodology used by Guerrero and Pérez-García (2021) for the analysis of the morphological variation of the mature stage of P. bullockii, all the steps of the analyses were performed using the same operator, and the specimens were re-digitized twice to avoid inter-observer variation in the data and to assess errors due to the digitization (Fruciano, 2016). After digitalization, a generalized Procrustes analysis (GPA) was performed in R v. 4.0.1 (R Core Team, 2020) using the geomorph v. 3.3.1 (Adams et al., 2021) package. Via the GPA, landmark coordinates were translated, rotated and scaled at unit centroid size (CS) to minimize the variation caused by size, orientation, location and rotation. The location of the semi-landmarks was optimized by minimizing the Procrustes distance between the reference and the target specimen, using throughout the function gpagen of geomorph v. 3.3.1 (Adams et al., 2021).
Principal component analyses (PCAs) were performed both for the original shape space (see Sect. S7 for more details) and the space of the data after size correction to remove the effect of the allometry (Figs. 5–8). Thus, for the latter, Procrustes coordinates were replaced by the residuals of the regression analysis of the Procrustes coordinates against CS (Mitteroecker et al., 2013). In this study, only the PCAs obtained from the Procrustes residuals were described in detail (Figs. 5–8). The number of significant PC scores kept was estimated using the broken-stick method (Jackson, 1993). Wireframe graphs and thin-plate spline deformation grids were aggregated to visualize shape variations according to the landmark arrangement. PCAs were carried out using geomorph v. 3.3.1 (Adams et al., 2021) and factoextra v. 1.0.6 (Kassambara and Mundt, 2020) packages.
Allometry was statistically examined by multivariate regression of Procrustes-aligned coordinates (dependent variable) against the logarithm to base 10 of CS values (independent variable). Permutation tests were requested with 10 000 runs to estimate the significance of the ontogenetic allometry in the sample (Good, 2000). The statistical significance of the regressions was tested with permutation tests against the null hypothesis of independence (Zelditch et al., 2012). These tests were performed through the geomorph v. 3.3.1 (Adams et al., 2021) package.
A multivariate normality was tested for each of the elements analysed. Specifically, the Shapiro–Wilk test was performed in R v. 4.0.1 (R Core Team, 2020), using the mvnormtest v. 0.1-9 (Jarek, 2012) package.
Likewise, a histogram was performed using the ggplot2 v. 3.3.2 (Wickham et al., 2021) package to visualize the size distribution of the different ontogenetic stages.
3.1 Qualitative approach
Ornamental pattern. All juvenile individuals analysed here display the same ornamentation pattern on the outer surface of the shell present in the adults of Pleurosternon bullockii (Fig. 1c–d), which consists of small, regular and clearly defined pits (e.g. NHMUK 48263, Fig. 2d; NHMUK 48347, Fig. 4c; Pérez-García and Ortega, 2011; Guerrero and Pérez-García, 2020, 2021). The size of the pitting relative to that of the plates remains constant regardless of the ontogenetic stage of the individuals. Likewise, the striations perpendicular to shell plates characteristic of Pleurosternidae are recognized in both juvenile (e.g. Fig. 1a) and adult individuals (Guerrero and Pérez-García, 2021). In the mature stage, the length of these striations is recognized as being variable, comparing several lateral areas of a single plate and different plates of the same specimen. The relative length and variation of the striations observed in the juveniles are compatible with those in the adults. In addition, some of the smallest individuals also possess thin and low midsagittal ridges anteroposteriorly developed along the dorsal surface of the carapace (NHMUK 48263a, Fig. 1e; NHMUK 48263e, Fig. 2c). Thus, these midsagittal ridges progressively decrease as ontogeny progresses, being absent in the mature stage. In fact, minor modifications in the carapace ornamentation pattern comparable with those recognized here for P. bullockii have been recognized as resulting from the ontogenetic variation in several species of extinct and extant turtles (Hay, 1908; Zangerl, 1953; de Broin, 1977; Somers et al., 2000; Danilov and Parham, 2008; Jansen and Klein, 2014; Cadena, 2015; Szczygielski et al., 2018).
Shell morphology. The preservation of only two of the small specimens allows inferences to be made about their almost complete carapace morphology (i.e. NHMUK 48262, Fig. 1a; NHMUK 48263, Fig. 2d). Both carapaces are slightly longer than wide. This condition is the same observed for the mature stage of Pleurosternon bullockii (Guerrero and Pérez-García, 2021). Thus, the relative dimensions of the carapace appear to be isometric throughout the ontogenetic development. None of the specimens preserve the complete plastron, but several partial and generally articulated plastra are identified (i.e. NHMUK 48263e, Fig. 2c; NHMUK 48354, Fig. 3a; NHMUK 48355, Fig. 3d; NHMUK 48346, Fig. 3f; NHMUK 48343, Fig. 4a; NHMUK48344, Fig. 4b; NHMUK 48347, Fig. 4c; NHMUK 48252, Fig. 4d). Most of them are exposed in ventral view, which allows for a detailed comparison with the plastron in the mature stage. The anterior plastral lobe, which is completely preserved in one individual (i.e. NHMUK 48343, Fig. 4a), is relatively narrower compared with that of the mature stage, being only slightly wider (i.e. the distance between the axillary notches) than long (i.e. the distance between the most anterior edge of the anterior lobe and the level of the axillary notches). In the medium-sized specimens of this species, recently identified as subadults (see Guerrero and Pérez-García, 2021), the width of the anterior lobe is about one and a half times its length (e.g. NHMUK OR 28433, see fig. 6D in Guerrero and Pérez-García, 2021). In adults, the width of the anterior lobe ranges from more than one and a half times to almost twice its length (e.g. NHMUK R 911 and NHMUK R 3413, see figs. 5A and 4F in Guerrero and Pérez-García, 2021). Thus, a growth pattern in relation to the relative proportions of this region is identified, which is evidence of an increase in its relative width. None of the small specimens preserve the complete posterior plastral lobe but only partial remains (i.e. NHMUK 48354, Fig. 3a; NHMUK 48355, Fig. 3d; NHMUK48343, Fig. 4a; NHMUK 48344, Fig. 4b; NHMUK 48252, Fig. 4d). Thus, the relative proportions of this region are unknown for most juveniles. The only exception is specimen NHMUK 48347 (Fig. 4c) since both the anal notch and the inguinal region are preserved; so they can be compared with those of specimens corresponding to other ontogenetic stages. The posterior plastral lobe of that juvenile individual (Fig. 3b) is almost two times wider (i.e. considering the distance between the inguinal notches) than long (i.e. taking into account the distance between the inguinal notch level and that of the anterior margin of the anal notch), whereas this region is slightly more than one and a half times wider than long in both subadults (e.g. NHMUK OR 40646, see fig. 5E in Guerrero and Pérez-García, 2021) and adults (e.g. NHMUK R 1889, see fig. 6B in Guerrero and Pérez-García, 2021). Therefore, an ontogenetic tendency corresponding to the increase in the relative length of the posterior plastral lobe is recognized.
Fontanelles and plate contacts. The preserved shells of the juveniles do not show fontanelles (Figs. 1–4), which supports the previous observation of Lapparent de Broin and Murelaga (1999). Thus, the juveniles of Pleurosternon bullockii do not have post-nuchal (i.e. NHMUK 48262, Fig. 1a; NHMUK 48263, Fig. 2d) or central plastral fontanelles (e.g. NHMUK 48343, Fig. 4a; NHMUK 48347, Fig. 4c). The juvenile stage is evidence that the contacts of some plates or shell regions (i.e. the contact of the peripherals and the pygal with the adjacent plates or the contact between the plastral bridges and the peripherals) were not very robust in this ontogenetic stage. Therefore, they are observed as disarticulated (e.g. NHMUK 48263a, Fig. 2a; NHMUK 48347, Fig. 4c). In the smallest specimens, a relatively long distal area of the ribs is free (e.g. NHMUK 48263a, Fig. 2a), suggesting the presence of costo-peripheral fontanelles. The presence of these structures is also evidenced by the absence of a serrated border on the distal margin of the costals, this region being unmineralized in the first stages of the ontogeny after hatching (Bona and Alcalde, 2009). Shell fontanelles are commonly identified as a juvenile feature and as an approach to know whether the individual has reached maturity both in extinct and extant turtle species belonging to clades in which adults lack these structures (Zangerl, 1939; de Broin, 1977; Gaffney, 1996; Bever, 2009; Jansen and Klein, 2014; Farke and Distler, 2015; Joyce, 2017; Shao et al., 2017, 2018; Limaverde et al., 2018; Anquetin and Püntener, 2020). Likewise, another character related to skeletogenesis that reveals the juvenile condition of the individuals studied here is the presence of a median suture strongly interdigitated between several plastral plates (e.g. that between both hyoplastra in NHMUK 48344, Fig. 4b). This condition has been identified as a typical character of immature individuals (Tong et al., 2004; Pritchard, 2008; Bona and Alcalde, 2009; Ferreira and Langer, 2013).
Nuchal plate. As previously described by some authors (Lydekker, 1889a, b; Lapparent de Broin and Murelaga, 1999; Milner, 2004), one noticeable difference between the juvenile and adult specimens of Pleurosternon bullockii corresponds to the morphology and relative dimensions of the nuchal plate. In the former (i.e. NHMUK 48262, Fig. 1a–b; NHMUK 48352, Fig. 2f), this plate is relatively shorter and broader compared with that of the adults (e.g. NHMUK OR 35574, see fig. 3A in Guerrero and Pérez-García, 2021). Specifically, the maximum width of the plate is more than one and a half (NHMUK 48352, Fig. 2f) or almost twice (NHMUK 48262, Fig. 1a–b) its length in the small specimens, whereas in adults, the nuchal length is less than one and a half its width in the vast majority of individuals (Guerrero and Pérez-García, 2021). The anterior margin of the nuchal in the juvenile specimens is very narrow, becoming up to more than five times less than the maximum width of the plate (NHMUK 48262, Fig. 1a–b). By contrast, this margin is quite wide in adults compared with those of the immature stage, ranging from more than two and a half times less than the maximum width of the plate (e.g. NHMUK 38733b, see fig. 2D in Guerrero and Pérez-García, 2021) to slightly more than one and a half times less than it (e.g. NHMUK OR 43621, see fig. 4A in Guerrero and Pérez-García, 2021).
Remarkable differences regarding the nuchal plate have also been observed in several extinct (Pérez-García, 2012; Shao et al., 2017; Gentry, 2018; Pérez-García, 2019) and extant turtles (Procter, 1922; Bona and Alcalde, 2009) during the ontogenetic development. These modifications generally imply variations in the relative size of this element, this plate becoming longer and narrower when the taxon grows (Shao et al., 2017; Pérez-García, 2019), as well as the presence of a more or less developed nuchal notch (Pérez-García, 2012; Gentry, 2018; Li et al., 2019). Thus, as in other turtle clades (e.g. Sinemydidae: Shao et al., 2017; Pan-Cheloniidae: Gentry, 2018), the variation in the relative proportions of the nuchal between the small and the adult specimens of P. bullockii is recognized as a result of the ontogenetic development. Regarding the nuchal notch, no significant differences have been observed in P. bullockii since it is not developed in adult individuals either, in contrast to some other pleurosternids (e.g. Dorsetochelys typocardium Seeley, 1869; see Pérez-García, 2014).
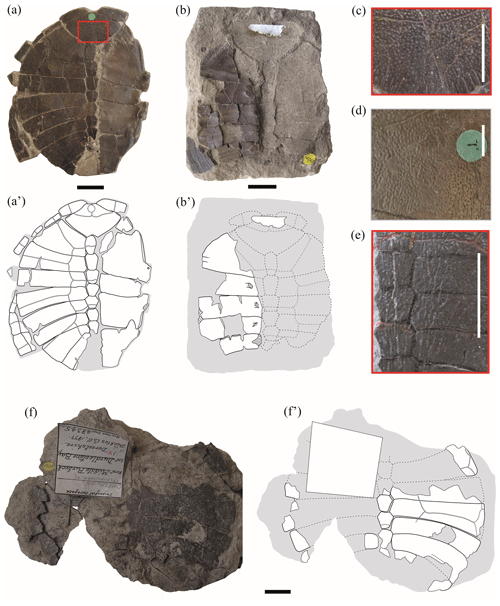
Figure 1Shells of juvenile specimens of Pleurosternon bullockii (Paracryptodira, Pleurosternidae), from the Berriasian (Early Cretaceous) of Swanage (Dorset, England) (a, b, f), and detail of the carapace ornamentation of juvenile (c, e) and adult (d) individuals. (a–c) NHMUK 48262, shell in dorsal (a) and ventral (b) views, as well as detail of the ornamentation of the nuchal (c). (d) Detail of the ornamentation of the nuchal of NHMUK OR 28618 (see fig. 3B in Guerrero and Pérez-García, 2021). (e) Detail of the ornamentation of the central area of the carapace of NHMUK 48263a (see Fig. 2a). (f) NHMUK 48345, in dorsal view. Scale bars equal 2 cm in (a), (b) and (f) and 1 cm in (c)–(e).
Neurals. The neural plates are other elements recognized here as being relatively variable when comparing the different ontogenetic stages of Pleurosternon bullockii. The complete or almost complete neural series is preserved in articulation in five of the small specimens (i.e. NHMUK 48262, Fig. 1a, b; NHMUK48263a, Fig. 2a; NHMUK 48263e, Fig. 2c; NHMUK48263, Fig. 2d; NHMUK 48264, Fig. 3c), whereas only a part of the series is preserved in articulation in the other carapaces (i.e. NHMUK 48345, Fig. 1f; NHMUK 48263c, Fig. 2b; NHMUK 48351, Fig. 2e; NHMUK 48354, Fig. 3a). In all these individuals, the relative proportions between the length and width of these plates have been identified as the most different feature in comparison with the adult specimens. In the former, these plates are, in general, relatively short and wide (e.g. NHMUK 48263a, Fig. 2a), whereas they are more elongated in the mature stage (e.g. NHMUK OR 35574, see fig. 3A in Guerrero and Pérez-García, 2021). As in P. bullockii, the relative proportions of the neural plates have been identified as variable during the ontogenetic development in other turtle species (Shao et al., 2017). In the literature, these shell elements have been described in the first stages of development as quadrangular plates (Gerlach, 2012). The fact that neurals undergo changes during ontogeny in their relative proportions and in their morphology is due to the relative longitudinal or lateral growth rates of each taxon (Gerlach, 2012). Thus, a greater growth rate in the longitudinal axis will lead to more elongated neurals, whereas a greater growth rate in the lateral axis will form broader and shorter elements. Likewise, in P. bullockii, a tendency in the decrease in the length width ratio towards the posterior region of the neural series has also been identified in most adults. Therefore, depending on the specimen and the region of the neural series, these plates will probably tend to follow a different grown pattern. Many of these plates in the mature stage of P. bullockii are relatively elongated compared with those of the juveniles except in the posterior region of the neural series. Therefore, in general, a greater longitudinal growth is determined for most of the elements of the neural series and a sub-equal growth rate in the posterior region of the neural row.
The hexagonal morphology is the predominant morphology reported in the immature and mature stages of P. bullockii (Guerrero and Pérez-García, 2021), indicating that the growth of most of these plates has been constrained by the adjacent bones (Gerlach, 2012). Likewise, juveniles also coincide with adults regarding the presence of a first neural divided transversely in some specimens (e.g. NHMUK 48263, Fig. 2d; CAMSM J5326, see fig. 2A in Guerrero and Pérez-García, 2021), as well as for having the same range of elements in the neural series (e.g. NHMUK 48263, Fig. 2d; see Guerrero and Pérez-García, 2021, for more details). Specifically, juveniles have been observed with seven and eight plates in the neural row and adults with a variation of seven to nine of these elements (Guerrero and Pérez-García, 2021).
Suprapygals. The study of the adult specimens of Pleurosternon bullockii allowed this taxon to be characterized as displaying two suprapygals (Pérez-García et al., 2015; Guerrero and Pérez-García, 2021). The first or anterior of these plates has been recognized in four juveniles (NHMUK 48263, Fig. 2d; NHMUK 48351, Fig. 2e; NHMUK 48354, Fig. 3a; NHMUK 48264, Fig. 3c), whereas the second one is not preserved in any of the specimens. The first suprapygal is almost complete in NHMUK 48263 (Fig. 2d) and NHMUK 48354 (Fig. 3a) but partially preserved in NHMUK 48264 (Fig. 3c) and NHMUK 48351 (Fig. 2e). As occurs in the mature stage, this plate can appear as an independent plate (NHMUK 48351, Fig. 2e) or be fused with the last neural, joining into a simple element (NHMUK 48263, Fig. 2d; NHMUK 48354, Fig. 3a). The preserved elements formed by the fusion of the last neural and first suprapygal range from slightly wider than long (NHMUK 48263, Fig. 2d) to less than one and a half times longer than wide (NHMUK 48354, Fig. 3a). By contrast, this element tends to be about twice as long as wide in the adult individuals (e.g. YPM VPPU 3364, see fig. 3D in Guerrero and Pérez-García, 2021). This difference is due to the greater growth in the longitudinal axis than in width as ontogeny advances. The morphology of this element in the small specimens coincides with those of the mature stage, being hexagonal and bilaterally symmetrical in all cases (e.g. NHMUK 48263, Fig. 2d; NHMUK 28619, see fig. S1.2F from appendix C in Guerrero and Pérez-García, 2021).
The absence of a preserved second suprapygal is justified by the non-ossified or slightly ossified union of this plate with the first suprapygal in the juvenile state, as happened with the peripheral plates in relation to the costals. In fact, embryological and ontogenetic studies have provided evidence that the suprapygal, pygal and peripheral plates are the last plates to ossify (Gilbert et al., 2001; Lima et al., 2011; Vieira et al., 2016). Consequently, the contact of these plates with other regions of the carapace showed great weakness in the immature stages, being easily disarticulated, to which its position in a peripheral region also contributes.
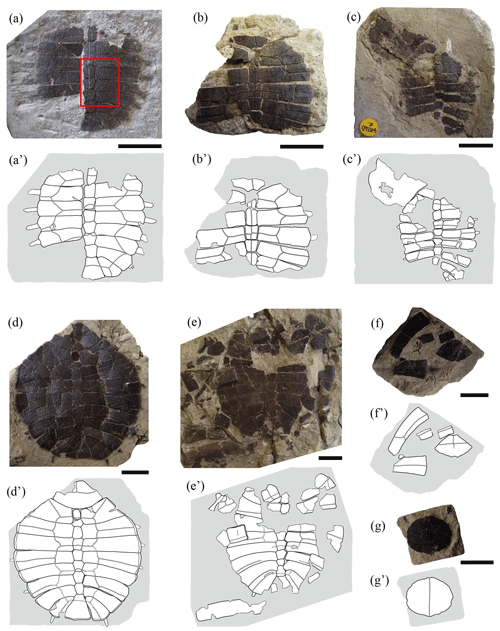
Figure 2Partial shells and isolated plates of juvenile specimens of Pleurosternon bullockii (Paracryptodira, Pleurosternidae), from the Berriasian (Early Cretaceous) of Swanage (Dorset, England). (a) NHMUK 48263a, in dorsal view. (b) NHMUK 48263c, in dorsal view. (c) NHMUK 48263e, in dorsal (carapace) and ventral (anterior plastral lobe) views. (d) NHMUK 48263, in dorsal view. (e) NHMUK 48351, in dorsal view. (f–g) NHMUK 48352, disarticulated plates of the carapace in dorsal view (f) and entoplastron in ventral view (g). Scale bars equal 2 cm.
Peripherals. The peripheral series is partially preserved in articulation in one of the juvenile specimens (NHMUK 48262, Fig. 1a). These plates are represented by relatively narrow osseous elements due to its proximal region being poorly ossified. Thus, a medial margin with small denticles for the contact with the costal series, recognized in the adult individuals of many turtles (including Pleurosternon bullockii), is absent. The peripherals of immature turtles mostly differ from those of the adults in their relative dimensions, being much longer than wide (Gilbert et al., 2001; Brinkman et al., 2013; Vieira et al., 2016; Schoch and Sues, 2020), as in the taxon studied here. Also, the first pair of peripherals in adults shows a well-developed contact with the first pair of costal plates (Guerrero and Pérez-García, 2021); however, this contact is absent in the juvenile stage (NHMUK 48262, Fig. 1a–b). The first pair of these plates of the immature individuals of P. bullockii taper towards the axial region (i.e. NHMUK 48262, Fig. 1a–b), being relatively close to each other, generating a notable emargination in the anterolateral margin of the carapace (even though a nuchal notch is absent). As in NHMUK 48262, the first peripheral plates of other taxa also modify their morphology between the juvenile and the adult stages, generating a different contour in the anterior carapace margin. Thus, the first peripheral plates of the adult specimens of the basal eucryptodiran Ordosemys leios Brinkman and Peng, 1993b generate a marked emargination in the anterior carapace margin, this being absent in the juveniles of this taxon. By contrast, the anterior margin of the first pair of peripherals of the juvenile individual of that species is straight, the anterior carapace margin lacking an emargination (Li et al., 2019, fig. 2). Based on the morphology of the first pair of peripherals of NHMUK 48262, Lapparent de Broin and Murelaga (1999) considered that this specimen was not attributable to P. bullockii, instead suggesting it were a form probably close to the North American Compsemydid genus of Compsemys Leidy, 1856, or belonging to it. However, the differences in the morphology and arrangement of these plates between this specimen and the adult individuals of P. bullockii are justified here as a result of the ontogenetic development, so the hypothesis put forward more than a century ago by Lydekker (1889a) proposing the attribution of a single taxon is supported here.
Vertebral and pleural scutes. As in other extinct and extant turtle taxa (e.g. de Broin, 1977; Joyce and Bell, 2004; Joyce, 2007; Pritchard, 2008; Jansen and Klein, 2014), notable differences in the relative proportions of the vertebral scutes have been observed in Pleurosternon bullockii, both comparing some juvenile specimens with the adults and comparing the juveniles with each other. The best-preserved scutes in the juveniles are the first three, and, therefore, it is in them that the greatest variation can be documented. The first vertebral scute of the small individuals differs from that of the adults both in the relative dimensions and in the morphology. This element is slightly more than two times wider than long in the immature stage (i.e. NHMUK 48262, Fig. 1a–b; NHMUK 48263, Fig. 2d), whereas its width is slightly less than twice its length in the adults (e.g. NHMUK R 3413, see fig. 4E in Guerrero and Pérez-García, 2021). As noted by Lapparent de Broin and Murelaga (1999) in the observation of the specimen NHMUK 48262 (Fig. 1a–b), the first vertebral of the small individuals is wide anteriorly, the anterior margin being two and a half times wider than the posterior. By contrast, the anterior border of the first vertebral of the adults is slightly narrower than the posterior (e.g. NHMUK OR 43621, see fig. 4A in Guerrero and Pérez-García, 2021). The posterolateral margin of the first vertebral of the juveniles is longer relative to the total length of the scute than that in the adults, that in the former reaching a length close to the maximum length of the vertebral (e.g. NHMUK 48262, Fig. 1a–b) but the posterolateral margin being two times less than the maximum length of the scute in the adults (e.g. NHMUK R 3413, see fig. 4E in Guerrero and Pérez-García, 2021). The second and third vertebrals of the smaller-sized juvenile specimens of P. bullockii are two and a half times wider than long (e.g. NHMUK 48263a, Fig. 2a; 48263e, Fig. 2c), whereas in the larger juveniles these are about one and a half times (NHMUK 48262, Fig. 1a–b; NHMUK 48263, Fig. 2d). In the mature stage, the relative proportions of both scutes vary from about two times wider than long (e.g. NHMUK R 5486, see fig. S1.2A from appendix C in Guerrero and Pérez-García, 2021) to one and a half times as wide as long (e.g. NHMUK OR 46317, see fig. 4C in Guerrero and Pérez-García, 2021). Thus, allometry is recognized considering the length width ratio of the P. bullockii specimens, a similar growth trend being recognized in other turtle taxa (Brinkman and Peng, 1993a; Valente et al., 2006; Shao et al., 2017; Pérez-García, 2019). However, it should be considered that the relative dimensions of these scutes in the mature stage of P. bullockii are very variable (Guerrero and Pérez-García, 2021), so the ontogeny is not the only factor that causes variation in the morphology of these scutes. Considering the relative dimensions of the vertebrals of the juveniles described here, the ontogenetic tendency of the pleural scutes is recognized as being the opposite (i.e. the width decreases relative to the length as ontogeny progresses). Likewise, the location of the distal margin of the pleurals has been recognized as being relatively variable in the mature stage, and this can be entirely located on the costal plates (e.g. CAMSM J5327, see fig. 2A in Guerrero and Pérez-García, 2021) or placed near the costo-peripheral sutures (e.g. NHMUK OR 28618, see fig. 2A in Guerrero and Pérez-García, 2021). This last condition coincides with that recognized in the juveniles studied here (e.g. NHMUK 48262, Fig. 1a; NHMUK 48263, Fig. 2d).
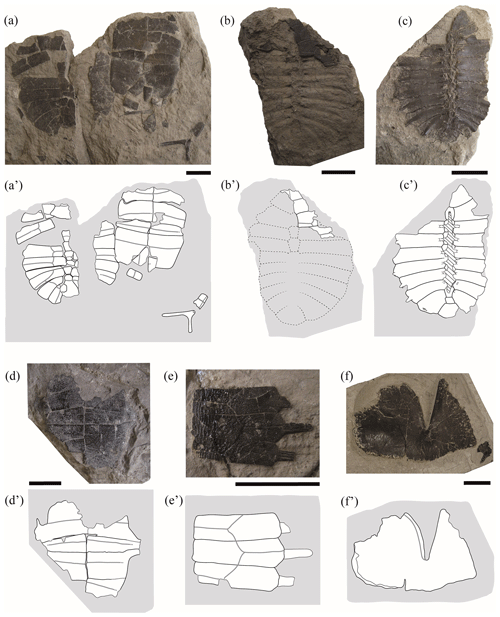
Figure 3Partial shells and isolated plates of juvenile specimens of Pleurosternon bullockii (Paracryptodira, Pleurosternidae), from the Berriasian (Early Cretaceous) of Swanage (Dorset, England). (a) NHMUK 48354, in dorsal (carapace) and ventral (plastron) views. (b–c) NHMUK 48264, in dorsal (b) and ventral (c) views. (d) NHMUK 48355, in ventral view. (e) NHMUK 48352, costal plates in dorsal view. (f) NHMUK 48346, hyoplastron in dorsal view. Scale bars equal 2 cm.
Entoplastron. Both the length and the width of the entoplastron are recognized as being sub-equal (this plate may even be slightly wider than long) for the entoplastra of the juvenile individuals of Pleurosternon bullockii (i.e. NHMUK 48352, Fig. 2g; NHMUK 48343, Fig. 4a). By contrast, the entoplastron of most adults is one and a half times wider than long or even wider, being rhombic (see Guerrero and Pérez-García, 2021). Therefore, the entoplastra of this species shows a positive allometry in their relative width as they grow, this ontogenetic change being compatible with that generally observed for other extinct and extant turtles (Procter, 1922; Püntener et al., 2017). There is also evidence of a relatively late development considering the entoplastra morphology due to the subadult specimens have an entoplastron more similar to that of the juveniles than that of the adults (e.g. NHMUK OR 28433, see fig. 6D in Guerrero and Pérez-García, 2021).
Mesoplastron. The mesoplastra of the small specimens of Pleurosternon bullockii differ in their morphology in relation to those of the adults. These pairs of plates are preserved in six juveniles, at least one mesoplastron being complete in two of them (NHMUK 48343, Fig. 4a; NHMUK 48347, Fig. 4c) and being partially preserved in the others (NHMUK48262, Fig. 1a; NHMUK 48354, Fig. 3a; NHMUK 48355, Fig. 3d; NHMUK 48344, Fig. 4b). As reported by some authors based on the observations of two of the juvenile specimens (NHMUK 48343, Fig. 4a; NHMUK 48347, Fig. 4c; Lydekker, 1889a; Lapparent de Broin and Murelaga, 1999), these plates are identified here as laterally wedged in all juveniles. By contrast, the mesoplastra of the adults and subadults display the same length across the entire plate. The elongation of the lateral region of the mesoplastra during ontogenetic development has also been described in the literature for other extinct turtles (e.g. Chisternon undatum Leidy, 1871; see Smith et al., 2017). For more details see Sect. 4.2 of the “Discussion”.
Gular scute. The gular and extragular scutes are exclusively known for one of the small specimens (NHMUK 48343, Fig. 4a). As previously reported by Lapparent de Broin and Murelaga (1999), the most remarkable difference in these scutes compared with those of the adults is that neither of the gulars of the juvenile reach the most anterior region of the entoplastron, whereas these scutes overlap the anterior area of this plate in all adults (Guerrero and Pérez-García, 2021). In addition, the posterior margin of the gulars of the juvenile specimen is curved, generating an anteriorly directed concavity (NHMUK 48343, Fig. 4a), but it is substraight in the adults (e.g. NHMUK 35772, see fig. 6E in Guerrero and Pérez-García, 2021). The condition observed in the subadults (e.g. NHMUK OR 40646, see fig. 5E in Guerrero and Pérez-García, 2021) is the same as that in the adults (e.g. NHMUK R 1890, see fig. 5F in Guerrero and Pérez-García, 2021). The relative proportions between the width and the length of the gular scutes of the juveniles, being about as wide as long, fit within the range previously observed for the adults and subadults (Guerrero and Pérez-García, 2021).
Plastral depressions for the contact with the pelvis. Lydekker (1889a) indicated that the visceral area of the plastron corresponding to the contact with the pelvis could differ between the juvenile and the adult individuals of Pleurosternon bullockii. The juvenile considered by Lydekker (1889a), NHMUK 48262 (Fig. 1a), continues to be the only immature specimen in which a partial xiphiplastron can be observed in dorsal view. For this ontogenetic stage, xiphiplastral depressions for the pelvic contact are not well-developed. By contrast, the adults present a slight hollow in the visceral surface of these plates (e.g. NHMUK R 4317, see fig. 5C in Guerrero and Pérez García, 2021), which has been interpreted both in P. bullockii and the other members of Pleurosternidae as being a facet for the mobile articulation with the pubis (Gaffney, 1979; Pérez-García and Ortega, 2011).
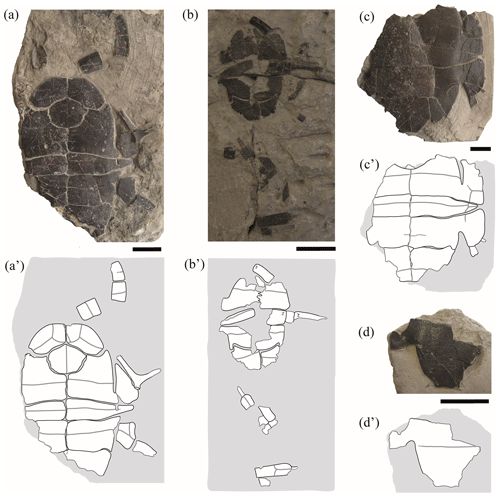
Figure 4Partial shells and isolated plates of juvenile specimens of Pleurosternon bullockii (Paracryptodira, Pleurosternidae), from the Berriasian (Early Cretaceous) of Swanage (Dorset, England). (a) NHMUK 48343, in ventral view. (b) NHMUK 48344, in ventral view. (c) NHMUK 48347, in ventral view. (d) NHMUK 48252, hypoplastron in ventral view. Scale bars equal 2 cm.
3.2 Quantitative approach
Shape variation. The PCA of the nuchal without the allometry effect (Fig. 5) shows an increase in the principal shape dimensions in relation to the PCA of the original shape of this plate (see Sect. S7). The first three principal components of the PCA of Procrustes residuals collectively account for 75 % of the total shape variance. The PC1 is principally associated with the location of the anterolateral and posterolateral contact of the nuchal plate of Pleurosternon bullockii. Thus, as positive loadings increase, the mid-lateral contact presents a greater distance regarding the posterior margin of the plate and vice versa. The PC2 is associated with the relative width and length of the nuchal, a wider and shorter plate representing the more positive values. The shape variance kept by the PC3 of this plate concerns the relative width of the anterior margin and the maximum width of the plate. In this context, a plate with a narrow anterior margin and that is relatively wider relative to the total length is observed for the positive values and vice versa for the negative values. In contrast to the PCA of the original shape space (see Sect. S7), the analysis of the data after size correction does not show a noticeable variation between the different ontogenetic stages. Therefore, the allometric factor plays a relatively significant role in the differentiation of the nuchal of the juvenile and the mature specimens of P. bullockii. As in the qualitative approach, the subadults are grouped with the adults in the quantitative analysis, showing the same condition regarding their morphology and relative dimensions.
The PCA corresponding to the morphogeometric data for the third vertebral scute of P. bullockii is illustrated in the space defined by the first three principal components (Fig. 6), which accounts for 87 % of the total shape variance of this scute. The PC1 is mainly associated with the variation in the relative length of the vertebral. As positive values increase, the scute is longer relative to its width and vice versa. The shape variance kept by the PC2 principally concerns the relative width of the scute and the location of the contact of the anterolateral and posterolateral margins regarding the lateral mid-point. Thus, the scute is wider and the contact of the anterior and posterior margins is farther from the lateral mid-point as positive values increase and vice versa for the negative values. The PC3 concerns the curvature degree of the anterior and posterior margins of the third vertebral, varying from a scute with straight anterior and posterior margins (positive scores) to a scute with curved anterior and posterior margins (negative scores). For this shell element, none of the axes of the PC separate the juveniles from the mature stage (i.e. subadult and adult individuals), so no significant morphological differences have been observed among ontogenetic stages. Regarding the PCA with the effect of the allometry (see Sect. S7 of the Supplement), the PC1 (i.e. the allometric axis) separates the third vertebral of the smaller-sized juveniles from that of the juveniles of greater size and the mature stage, which is compatible with the qualitative observations (see above). Thus, as previously suggested (see Sect. 3.1 of the “Results”), probably the third vertebral scute already reached the condition observed for the adults in the immature stage. In addition, as observed for the matures, noticeable variations in the scute morphology have also been demonstrated among the smaller juveniles, and there is evidence from the immature stages of the ontogeny of a relatively high inter-individual variation.
The first two principal components related to the analysis of the anterior plastral lobe outline of P. bullockii account for 81 % of the total variance in the dataset (Fig. 7). The PC1 concerns the relative width of the posterior region of the anterior plastral lobe (i.e. the distance between the axillary notches), being greater as positive values increase and vice versa for negative values. The shape variance kept by the PC2 is mainly associated with the variation in the lateral margin outline, varying from an anterior plastral lobe with rounded lateral margins (negative scores) to an anterior lobe with straight lateral margins (positive scores). In contrast to the PCA in which the allometry factor is considered (see Sect. S7), the PCA without the effect of that factor does not differentiate the three ontogenetic stages (i.e. juvenile, subadult and adult) previously observed by the qualitative approach. In this context, the morphology of the juvenile individuals falls within the variation of those of the mature stage (i.e. the adult individuals) in both principal components. In this analysis, the variation among the individuals is influenced to a greater extent by the inter-individual variation than by the ontogenetic one. In this case, the specimens of the mature stage, which have similar sizes, present high morphologic variation in this area. Therefore, this variation could be due to this region is highly polymorphic as generated by sexual dimorphism. Likewise, the subadult specimens do not differ significantly from some adults such that the variation resulting from ontogeny loses relevance in the total percentage of the variation in the anterior plastral lobe.
The first two principal components of the PCA accumulate 87 % of the total shape variance for the entoplastron of P. bullockii (Fig. 8). The PC1 mainly concerns the roundness and the degree of wedging of the lateral margins and the relative length of the plate. As positive values increase, the entoplastral lateral margins are more rounded, showing less acute lateral vertices, resulting in a larger plate and vice versa. The PC2 eigenvalue is associated with the variation in the position of contact between the anterolateral and posterolateral entoplastral margins, as well as the curvature degree of the anterolateral margins. Thus, positive values are associated with a lateral contact placed more posteriorly on the plate and the presence of straighter anterolateral margins, and vice versa for the negative values. In this case, the PC1 separates the three ontogenetic stages recognized through the qualitative approach (see above), as in the PCA with the allometric effect (see Sect. S7). The ontogeny is also not recognized as the principal factor of variation (Fig. 8). In fact, the inter-individual variation has more relevance for the morphological variation of this element than the ontogenetic development. Thus, remarkable differences among adults can be observed (e.g. between NHMUK R 6912 and NHMUK 46832, see figs. 6C and S1.5A from appendix C in Guerrero and Pérez-García, 2021). Therefore, as occurs for the other shell elements analysed quantitatively, the morphologic variation product of the ontogeny loses importance against other types of intraspecific variation such as the inter-individual one.
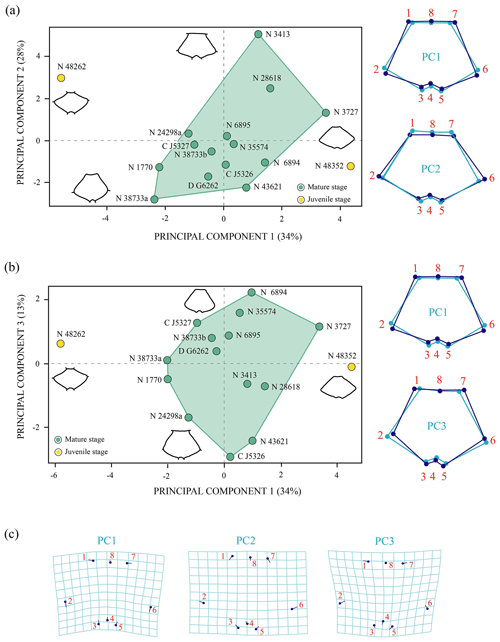
Figure 5Shape differences of the nuchal of Pleurosternon bullockii (Paracryptodira, Pleurosternidae), taking into account specimens from the Berriasian (Early Cretaceous) of Swanage (Dorset, England). (a–b) Principal component analysis (PCA). Wireframes indicate the main shape changes in each PC; the light blue concerns the average shape, whereas the dark blue is the shape variance related to the extreme of variation along the axis. (c) Transformation grids to visualize shape deformation relative to the first three principal components. Abbreviations: C, CAMSM; D, DORCM; N, NHMUK.
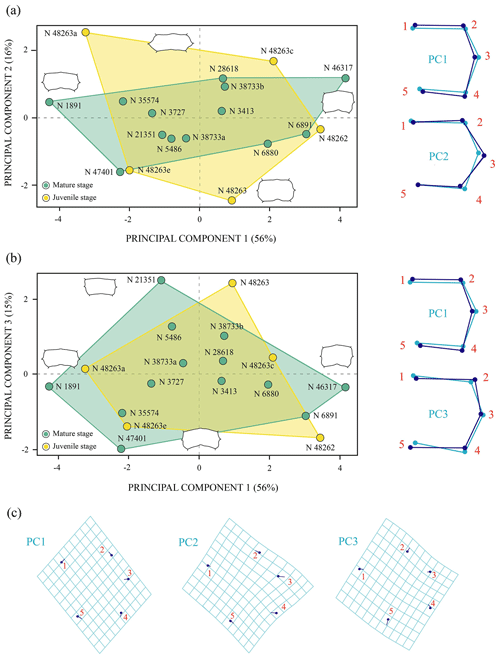
Figure 6Shape differences of the third vertebral scute of Pleurosternon bullockii (Paracryptodira, Pleurosternidae), taking into account specimens from the Berriasian (Early Cretaceous) of Swanage (Dorset, England). (a–b) Principal component analysis (PCA). Wireframes indicate the main shape changes in each PC; the light blue concerns the average shape, whereas the dark blue is the shape variance related to the extreme of variation along the axis. (c) Transformation grids to visualize shape deformation relative to the first three principal components. Abbreviation: N, NHMUK.
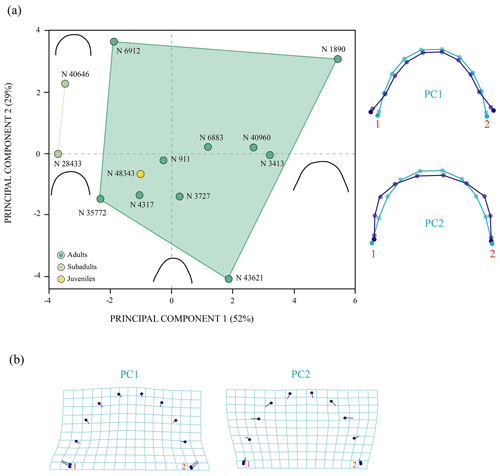
Figure 7Shape differences of the anterior plastral lobe of Pleurosternon bullockii (Paracryptodira, Pleurosternidae), taking into account specimens from the Berriasian (Early Cretaceous) of Swanage (Dorset, England). (a) Principal component analysis (PCA). Wireframes indicate the main shape changes in each PC; the light blue concerns the average shape, whereas the dark blue is the shape variance related to the extreme of variation along the axis. (b) Transformation grids to visualize shape deformation relative to the first three principal components. Abbreviation: N, NHMUK.
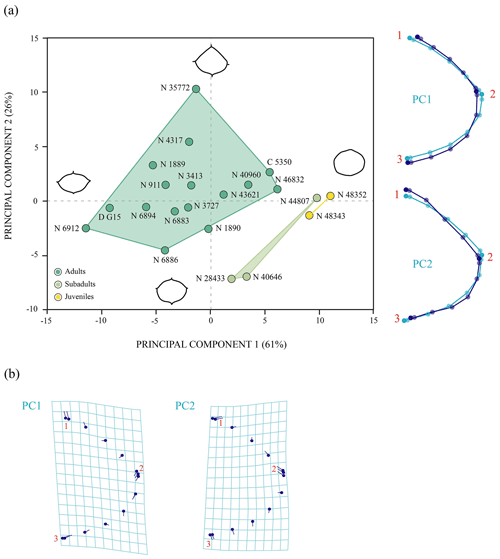
Figure 8Shape differences of the entoplastron of Pleurosternon bullockii (Paracryptodira, Pleurosternidae), taking into account specimens from both the Tithonian (Late Jurassic) and Berriasian (Early Cretaceous) of England. (a) Principal component analysis (PCA). Wireframes indicate the main shape changes in each PC; the light blue concerns the average shape, whereas the dark blue is the shape variance related to the extreme of variation along the axis. (b) Transformation grids to visualize shape deformation relative to the first three principal components. Abbreviations: C, CAMSM; D, DORCM; N, NHMUK.
Normality tests. Shapiro–Wilk tests indicated a normal distribution (p>0.05) for the nuchal plate and the third vertebral scute of Pleurosternon bullockii (see Sect. S8a and b for more details). For the shell elements (i.e. anterior plastral lobe and entoplastron, see Sect. S8c and d for more details) without a normal distribution (p<0.05), the descriptions of parametric analyses (i.e. regression; Fig. 9c–d) have not been added for these elements since the validity of the parametric test cannot be confirmed.
Allometry. Both the nuchal plate and the third vertebral scute of Pleurosternon bullockii (Fig. 9a–b) are influenced by allometry (p value: <0.05). The former has a higher percentage of the shape variation explained by size (53 %) than the vertebral scute (20 %).
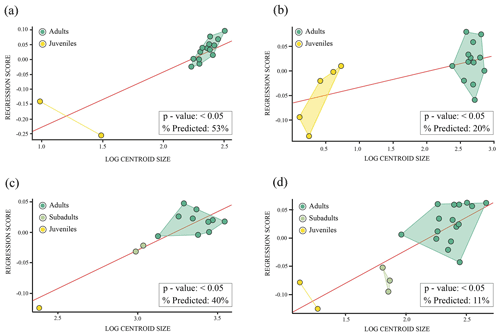
Figure 9Linear regression between Procrustes coordinates (dependent variable) and logged-centroid size values (independent variable) of the shell elements of Pleurosternon bullockii (Paracryptodira, Pleurosternidae), from the Tithonian (Upper Jurassic) and Berriasian (Lower Cretaceous) of England. (a) Nuchal. (b) Third vertebral scute. (c) Anterior plastral lobe. (d) Entoplastron.
4.1 Systematic attribution and ontogenetic implications
Most of the characters described for the juvenile turtle individuals studied here, from the British Lower Cretaceous record, coincide with those considered in the recently published amended diagnosis of Pleurosternon bullockii (Guerrero and Pérez-García, 2021), sharing a unique character combination with this taxon. In fact, adult individuals of this species were also found in the Purbeck Limestone Group of Swanage (Dorset), including the type material. All diagnostic characters of P. bullockii not coincident with the states observed here for the juvenile specimens are related to their relative dimensions (i.e. width of the posterior margin of the first peripheral about two times less than the anterior one; first to third vertebral scutes between three and a half times to two times wider than the pleural scutes; first to third pleural scutes relatively long in relation to their width, being always longer than wide; medial length of the mesoplastra being similar to the lateral one). These differences in the proportions of these shell elements have been recognized, both in this study (see above) as well as in the literature, as being dependent on the ontogenetic development. Therefore, taxonomic variation is rejected as a causative factor for the difference of these character states between the mature and the juvenile individuals. Likewise, other characters not included in the diagnosis of P. bullockii and that also differ between the adult specimens of this species and the juvenile individuals studied here (e.g. presence/absence of fontanelles or the plastral depressions for the contact with the pelvis) are also justified as being subject to ontogenetic variation (see Sect. 3.1 of the “Results”).
Based on the variation recognized between the juvenile specimens and the individuals corresponding to the mature stage (see above), as well as the difference in the size of the specimens (i.e. small, medium and large), two ontogenetic stages (i.e. juvenile and adult; for more details see Sect. S9) have been recognized for the carapace of P. bullockii and three (i.e. juvenile, subadult and adult; for more details see Sect. S10) for its plastron. Ontogenetic variation does not represent in all cases the primary source of intraspecific variation in this taxon. Thus, for some elements and regions (i.e. the morphology of the carapace and plastron contour, and that of several plates and scutes including some neurals, the first suprapygal, some vertebral scutes and the entoplastron), the variation observed among the adults is identified as more significant than that observed between the different ontogenetic stages. Therefore, a high inter-individual variation is demonstrated for the P. bullockii species, which is in accordance with that recognized in previous works (see Guerrero and Pérez-García, 2021, and references therein). Sexual dimorphism has been excluded as one of the main factors causing the variations for P. bullockii described in this study, on the one hand, because the differences observed between the specimens of P. bullockii are not commonly recognized in the literature as sexually dimorphic (Pritchard, 1979, 2008). On the other hand, due to different sizes having been recognized, sexual morphotypes of P. bullockii appear to attain approximately the same maximum size (Guerrero and Pérez-García, 2021).
4.2 Comparison of the ontogenetic development of Pleurosternon bullockii with that of other pleurosternids and other paracryptodires
The ontogenetic pattern of development in Pleurosternidae is poorly known due to the limited availability of juvenile specimens of this clade. So far, Pleurosternon bullockii stands out for being the pleurosternid with the highest number of immature specimens. Scarce immature specimens are so far known for other representatives of this clade, all of them belonging to North American taxa (Gaffney, 1979; Brinkman et al., 2000). These are a specimen of Glyptops plicatulus Cope, 1877 (DNM 992, Gaffney, 1979, fig. 11) and two individuals previously attributed to Dinochelys whitei Gaffney, 1979 (DNM 993, Gaffney, 1979, fig. 10; BYU 13099, Brinkman et al., 2000, figs. 1, 2), this last attribution having recently been proposed as doubtful (Brinkman, 2018).
The immature stage of G. plicatulus and putatively of Di. whitei coincide with that of P. bullockii in the presence of sagittal ridges in the carapace surface, and, similar to the latter, in the juvenile specimens of the North American taxa, these ridges disappear in the adult stage (Gaffney, 1979; Brinkman et al., 2000; Brinkman, 2018). The more significant difference in these ridges between juveniles of P. bullockii and those of G. plicatulus and Di. whitei is their direction of development. In these latter taxa, the ridges are extended forwards and outwards from a midpoint on the posterior edge of the neurals (Brinkman et al., 2000), whereas in P. bullockii these are anteroposteriorly developed. Variations in the ornamentation of the carapace surface have also been reported for some taxa of the sister group of Pleurosternidae (i.e. Baenidae: for Baena arenosa Leidy 1870 and Chisternon undatum), probably a product of their ontogenetic development (Smith et al., 2017). Specifically, the smaller subadults (e.g. Baena arenosa; Smith et al., 2017, fig. 11) are described with a triple ridge corresponding to three parallel elevations on the dorsal surface of the neural row being less pronounced in the largest subadults and absent in the adults.
Slight differences related to the relative dimensions of the shell have been observed here between the juvenile and adult individuals of P. bullockii, such as those in G. plicatulus and Di. whitei. The pleurosternid G. plicatulus also shows a variation in the relative dimensions of its carapace (i.e. the carapace of the juvenile being narrower than the adult), as well as in the posterior plastral lobe (i.e. this region being narrower in the juveniles than in the adults). The anterior plastral lobe is not completely preserved in any of the juvenile individuals of G. plicatulus currently known; therefore, its morphology, as well as its relative dimensions, cannot be determined in this taxon. In contrast to P. bullockii and G. plicatulus, no variation has been observed in Di. whitei regarding the relative proportions of the carapace or the plastron between the putative juveniles and the adult individuals.
The ontogenetic pattern regarding the variation in the relative proportions of the neural series has also been observed in G. plicatulus and putatively in Di. whitei. As in P. bullockii, in both of the North American taxa it appears that the growth rate of these plates is greater in the longitudinal direction than the lateral.
Regarding the peripheral plates, the putative juveniles of Di. whitei present a similar condition to the juveniles of P. bullockii, both taxa being characterized as having very narrow peripherals forming a thin ring in the shell periphery (Brinkman et al., 2000). By contrast, the peripherals of the immature stage of G. plicatulus are similar (i.e. both in morphology and considering the relative dimensions) to those observed in the mature stage, this taxon being evidence of an earlier development of these plates during the ontogeny than P. bullockii and Di. whitei.
As in P. bullockii, the relative dimensions and the morphology of the vertebral scutes of both North American taxa, as well as the pleural scutes of G. plicatulus, are also conditioned by the ontogenetic development. Thus, the three species present an allometric decrease in the relative width of the vertebral scutes as ontogeny progresses (Brinkman et al., 2000). However, in the case of the pleurals, G. plicatulus and P. bullockii do not present the same direction of growth. Specifically, in the latter, these scutes also have an allometric decrease in their relative width, whereas, in G. plicatulus, they present an increase in their relative width.
As in P. bullockii, the relative size and morphology of the entoplastron of G. plicatulus and putatively that of Di. whitei are clearly dependent of the ontogenetic development. The entoplastron of the juvenile of the three taxa is quite different to that of the adults. The immature specimens of P. bullockii and G. plicatulus also share the condition that the relative proportions of mesoplastra change during the ontogenetic development. Nevertheless, in the juveniles of G. plicatulus there is no wedging in these plates (nor lateral or medial), in contrast to the lateral wedging recognized for all juveniles of P. bullockii. Likewise, a similar variation to that observed in the mesoplastron of the juveniles of P. bullockii has been recognized in Baenidae (specifically in Chisternon undatum; see Smith et al., 2017). In the latter, the subadults are differentiated from the adults based on their noticeable narrowing of mesoplastra, resulting in a shorter medial articulation between both plates (Smith et al., 2017).
Some notable differences related to the ontogenetic development have also been observed among the juveniles of P. bullockii and those of G. plicatulus and Di. whitei. The most notable is the presence of fontanelles in the juvenile individuals of these North American taxa being present in both the carapace and the plastron (see Gaffney, 1979; Brinkman et al., 2000). The lack of fontanelles in the juvenile specimens of P. bullockii is evidence that the shell of this taxon has an earlier development for this character than the other pleurosternids. In fact, as indicated by Brinkman et al. (2000), Di. whitei is the pleurosternid in which the shell acquires the adult condition in a more advanced ontogenetic stage since – although the shell of Di. whitei is similar in size to juveniles of G. plicatulus and P. bullockii – its fontanelles (absent in P. bullockii) are much more developed than those of G. plicatulus.
The nuchal plate of the juveniles of G. plicatulus and Di. whitei is similar to the morphology of their mature stage; only some subtle differences regarding the relative proportions of the plate have been observed. This can indicate that the nuchal acquired the morphology of the adult stage in a more juvenile ontogenetic stage in the North American taxa.
Gular scutes are preserved in the putative juveniles of Di. whitei but not in those of G. plicatulus, in which they do not show significant differences with those of the adults. Thus, this lack of an evident variation among ontogenetic stages implies an early development of the morphology and relative dimensions of the gular scutes.
Thus, based on these comparisons, there is evidence of different ontogenetic trajectories for the members of Pleurosternidae, in which both juvenile and adult individuals are known. As previously observed among representatives of other turtle groups (e.g. Sinemydidae, Proterochersidae or Baenidae; Shao et al., 2017; Smith et al., 2017; Szczygielski et al., 2018; Li et al., 2019), in shell elements that are ontogenetically dependent, the magnitude of shape change, as well as the growth rate, varies at the specific level. Therefore, in general, the ontogenetic development of P. bullockii is not shared with that known for any other pleurosternid.
4.3 Palaeoecological implications
Most British specimens of Pleurosternon bullockii known so far come from the Purbeck Limestone Group of Swanage, Dorset (Milner, 2004). They were found in different levels (Milner, 2004; West, 2013). According to Milner (2004), most of the small specimens come from the Mammal Bed (DB 83), which corresponds to the deposits of a shallow freshwater lake (Sweetman et al., 2018). Instead, most of the adults were found in the Intermarine Member (DB 112–145), described as deposits of lagoons that fluctuated from marine to freshwater conditions (Wright et al., 1997). Only a few shell remains of adult specimens of P. bullockii have been registered in other levels of the Purbeck Limestone Group but also different from those where the juveniles were found (i.e. the Cinder Member, DB 111, and the Crocodile Bed, DB 221; see Milner, 2004). Thus, all these specimens were located in the Middle Purbeck Unit (Milner, 2004), and, despite all these adults being situated in slightly more recent stratigraphic levels than that where the juveniles were found, adult specimens of this species were also recognized at older levels outside the Purbeck Limestone Group (i.e. in the Portland Group; see Guerrero and Pérez-García, 2020). Therefore, the fact that the juvenile specimens were found in a different level than the adults probably suggests that they had a different lifestyle than the adults to maintain optimal growth rates. The shift in habitat utilization during the life cycles is widely known for many extant freshwater and marine turtle species (Snover, 2002; Myers et al., 2007; Castellano et al., 2008; Paterson et al., 2014; Pate and Salmon, 2017). It has been suggested that specific habitats can have a strong influence on the survival of the immature stage of some turtle species due to favourable environmental conditions, protection from predators or food availability (Snover, 2002; Paterson et al., 2014). Therefore, it is possible that the juvenile individuals of P. bullockii used the environment of the shallow lake to maximize growth rates while minimizing mortality risks during the early stages of their development.
Several small specimens of pleurosternid turtles from the British Purbeck Limestone Group (Berriasian, Lower Cretaceous) are analysed in detail. Most of them were unpublished specimens despite having been found in the 19th century. Due to the poor knowledge about these individuals so far available, their systematic attribution was doubtful, having been considered both as juveniles of Pleurosternon bullockii, also found in the Purbeck Limestone Group but at different levels, and as members of a new undefined taxon. This analysis allows us to justify their identification as juvenile individuals of P. bullockii.
The ontogenetic pattern of development of Pleurosternidae was poorly known due to the limited availability of juvenile specimens of the clade. However, the presence of several well-preserved and articulated individuals of different sizes has enabled us to characterize the shell morphology corresponding to the immature stage of P. bullockii, as well as to identify modifications experienced throughout the ontogenetic development, from that stage to the subadult and adult stages. Ontogenetic variation does not always constitute the principal source of intraspecific variation in P. bullockii. For several shell elements or regions, inter-individual variation has more influence on the morphological changes than the ontogenetic one. Thus, evidence of a high inter-individual variation is found in P. bullockii. The comparative study of the ontogenetic series of P. bullockii with those of other members of Pleurosternidae has revealed different ontogenetic trajectories for the few members of the group in which juveniles are known. The shell elements that are ontogenetically variable, the magnitude of the shape changes and the growth rate vary at the specific level, as occurs among the representatives of other clades of turtles.
The juvenile specimens of P. bullockii were found in different levels of the Purbeck Limestone Group than those where the adults were identified, corresponding to a different environment (i.e. shallow and freshwater lakes vs. lagoons that fluctuated from marine to freshwater conditions). This suggests the possibility that the juvenile individuals had a different lifestyle from that of the adults to maximize growth rates while minimizing mortality risks during the early stages of their development.
The specimens studied here are deposited in the Natural History Museum (London, United Kingdom).
The supplement related to this article is available online at: https://doi.org/10.5194/fr-24-357-2021-supplement.
AG analysed the data, wrote the paper and prepared figures. APG analysed the data, reviewed drafts of the paper and approved the final draft.
The contact author has declared that neither they nor their co-author has any competing interests.
Publisher’s note: Copernicus Publications remains neutral with regard to jurisdictional claims in published maps and institutional affiliations.
The authors thank Sandra Chapman (NHMUK) for access to the material studied here. Also, we would like to thank the staff of the CAMSM, DORCM, OUMNH and UMZC for access to the comparative material used in this study, as well as Vanessa R. Rhue (YPM) for providing us with pictures of two of the specimens (YPM VPPU 3363 and YPM VPPU 3364) of the comparative material. The authors also thank the editor Torsten Scheyer and the reviewers Don Brinkman (Royal Tyrrell Museum of Palaeontology, Canada), Igor Danilov (Zoological Institute, Russian Academy of Sciences, Russia) and Tomasz Szczygielski (University of Warsaw and Polish Academy, Poland) for their useful comments and their constructive reviews.
This research received support from several SYNTHESYS Projects (European Commission).
This paper was edited by Torsten Scheyer and reviewed by Tomasz Szczygielski, Igor Danilov, and Don Brinkman.
Adams, D., Collyer, M., and Kaliontzopoulou, A.: Geomorph: Software for geometric morphometric analyses, R package version 3.2.1, 2021.
Anquetin, J. and Püntener, C.: A new species of the large-headed coastal marine turtle Solnhofia (Testudinata, Thalassochelydia) from the Late Jurassic of NW Switzerland, PeerJ, 8, e9931, https://doi.org/10.7717/peerj.9931, 2020.
Bever, G. S.: Postnatal ontogeny of the skull in the extant North American turtle Sternotherus odoratus (Cryptodira: Kinosternidae), B. Am. Mus. Nat. Hist., 330, 1–97, https://doi.org/10.1206/330.1, 2009.
Bona, P. and Alcalde, L.: Chondrocranium and skeletal development of Phrynops hilarii (Pleurodira: Chelidae), Acta Zool., 90, 301–325, https://doi.org/10.1111/j.1463-6395.2008.00356.x, 2009.
Brinkman, D. B.: Two new paracryptodire turtles from Western Canada, in: Turtle Evolution Symposium, 26–27 May 2018, Tokyo, Japan, 15–16, 2018.
Brinkman, D. B. and Peng, J.-H.: New material of Sinemys (Testudines, Sinemydidae) from the Early Cretaceous of China, Can. J. Earth Sci., 30, 2139–2152, https://doi.org/10.1139/e93-185, 1993a.
Brinkman, D. B. and Peng, J-H.: Ordosemys leios, n.gen., n.sp., a new turtle from the Early Cretaceous of the Ordos Basin, Inner Mongolia, Can. J. Earth Sci., 30, 2128–2138, https://doi.org/10.1139/e93-184, 1993b.
Brinkman, D. B., Stadtman, K., and Smith, D.: New material of Dinochelys whitei Gaffney, 1979, from the Dry Mesa Quarry (Morrison Formation, Jurassic) of Colorado, J. Vertebr. Paleontol., 20, 269–274, https://doi.org/10.1671/0272-4634(2000)020[0269:NMODWG]2.0.CO;2, 2000.
Brinkman, D. B., Yuan, C.-X., Ji, Q., Li, D.-Q., and You, H.-L.: A new turtle from the Xiagou Formation (Early Cretaceous) of Changma Basin, Gansu Province, P. R. China, Palaeobio. Palaeoenv., 93, 367–382, https://doi.org/10.1007/s12549-013-0113-0, 2013.
de Broin, F.: Contribution à l'étude des Chéloniens continentaux du Crétacé et du Tertiaire de France, Mémoires de Muséum National d'Histoire Naturelle, 38, 1–366, 1977.
Cadena, E.: A global phylogeny of Pelomedusoides turtles with new material of Neochelys franzeni, Schleich 1993 (Testudines, Podocnemididae) from the middle Eocene, Messel Pit, of Germany, PeerJ, 3, e1221, https://doi.org/10.7717/peerj.1221, 2015.
Castellano, C. M., Behler, J. L., and Ultsch, G. R.: Terrestrial movements of hatchling wood turtles (Glyptemys insculpta) in agricultural fields in New Jersey, Chelonian Conserv. Bi., 7, 113–118, https://doi.org/10.2744/CCB-0677.1, 2008.
Cope, E. D.: On reptilian remains from the Dakota Beds of Colorado, P. Am. Philos. Soc., 17, 193–196, 1877.
Danilov, I. G. and Parham, J. F.: A reassessment of some poorly known turtles from the Middle Jurassic of China, with comments on the antiquity of extant turtles, J. Vertebr. Paleontol., 28, 306–318, https://doi.org/10.1671/0272-4634(2008)28[306:AROSPK]2.0.CO;2, 2008.
Farke, C. M. and Distler, C.: Ontogeny and abnormalities of the tortoise carapace: A computer tomography and dissection study, Salamandra, 51, 231–244, 2015.
Ferreira, G. S. and Langer, M. C.: A pelomedusoid (Testudines, Pleurodira) plastron from the Lower Cretaceous of Alagoas, Brazil, Cretaceous Res., 46, 267–271, https://doi.org/10.1016/j.cretres.2013.10.001, 2013.
Fish, J. F. and Stayton, C. T.: Morphological and mechanical changes in juvenile red-eared slider turtle (Trachemys scripta elegans) shells during ontogeny, J. Morphol., 275, 391–397, https://doi.org/10.1002/jmor.20222, 2014.
Fruciano, C.: Measurement error in geometric morphometrics, Development Genes and Evolution, 226, 139–158, https://doi.org/10.1007/s00427-016-0537-4, 2016.
Gaffney, E. S.: The Jurassic turtles of North America, B. Am. Mus. Nat. Hist., 162, 93–135, 1979.
Gaffney, E. S.: The postcranial morphology of Meiolania platyceps and a review of the Meiolaniidae, B. Am. Mus. Nat. Hist., 229, 1–166, 1996.
Gentry, A. D.: Prionochelys matutina Zangerl, 1953 (Testudines: Pan-Cheloniidae) from the Late Cretaceous of the United States and the evolution of epithecal ossifications in marine turtles, PeerJ, 6, e5876, https://doi.org/10.7717/peerj.5876, 2018.
Gerlach, J.: Skeletal ontogeny of Seychelles giant tortoises (Aldabrachelys/Dipsochelys), Sci. Res. Essays, 7, 1083–1099, https://doi.org/10.5897/SRE11.2019, 2012.
Gilbert, S. F., Loredo, G. A., Brukman, A., and Burke, A. C.: Morphogenesis of the turtle shell: the development of a novel structure in tetrapod evolution, Evolution and Development, 3, 47–58, https://doi.org/10.1046/j.1525-142x.2001.003002047.x, 2001.
Good, P. I.: Permutation Tests: A practical guide to resampling methods for testing hypotheses, Springer Series in Statiastics, Springer-Verlag, New York, 270 pp., 2000.
Guerrero, A. and Pérez-García, A.: On the validity of the British Upper Jurassic turtle “Pleurosternon portlandicum” (Paracryptodira, Pleurosternidae), J. Iber. Geol., 46, 419–429, https://doi.org/10.1007/s41513-020-00136-x, 2020.
Guerrero, A. and Pérez-García, A.: Morphological variability and shell characterization of the European uppermost Jurassic to lowermost Cretaceous stem turtle Pleurosternon bullockii (Paracryptodira, Pleurosternidae), Cretaceous Res., 125, 104872, https://doi.org/10.1016/j.cretres.2021.104872, 2021.
Gunz, P. and Mitteroecker, P.: Semilandmarks: a method for quantifying curves and surfaces, Hystrix, 24, 103–109, https://doi.org/10.4404/hystrix-24.1-6292, 2013.
Hay, O. P.: The fossil turtles of North America, Carnegie Institution of Washington, Washington D.C., United States, 568 pp., 1908.
Jackson, D. A.: Stopping rules in principal components analysis: a comparison of heuristical and statistical approaches, Ecology, 74, 2204–2214, https://doi.org/10.2307/1939574, 1993.
Jansen, M. and Klein, N.: A juvenile turtle (Testudines, Eucryptodira) from the Upper Jurassic of Langenberg Quarry, Oker, Northern Germany, Palaeontology, 57, 743–756, https://doi.org/10.1111/pala.12085, 2014.
Jarek, S.: mvnormtest: Normality test for multivariate variables, R package version 0.1-9, 2012.
Joyce, W. G.: Phylogenetic Relationships of Mesozoic Turtles, B. Peabody Mus. Nat. Hist., 48, 3–102, https://doi.org/10.3374/0079-032X(2007)48[3:PROMT]2.0.CO;2, 2007.
Joyce, W. G.: A review of the fossil record of basal Mesozoic turtles, B. Peabody Mus. Nat. Hist., 58, 65–113, https://doi.org/10.3374/014.058.0105, 2017.
Joyce, W. G. and Bell, C. J.: A review of the comparative morphology of extant testudinoid turtles (reptilia: testudines), Asiatic herpetological research, 10, 53–109, 2004.
Joyce, W. G., Rollot, Y., and Cifelli, R. L.: A new species of baenid turtle from the Early Cretaceous Lakota Formation of South Dakota, Foss. Rec., 23, 1–13, https://doi.org/10.5194/fr-23-1-2020, 2020.
Kassambara, A. and Mundt, F.: factoextra: Extract and Visualize the Results of Multivariate Data Analyses, R package version 1.0.7, 2020.
Lapparent de Broin, F. de and Murelaga, X.: Turtles from the Upper Cretaceous of Laño (Iberian Peninsula), Estudios del Museo de Ciencias Naturales de Álava, 14, 135–211, 1999.
Larson, P. M.: Chondocranial development in larval Rana sylvatica (Anura: Ranidae): morphometric analysis of cranial allometry and ontogenetic shape change, J. Morphol., 252, 131–144, https://doi.org/10.1002/jmor.1095, 2002.
Leidy, J.: Notices of extinct Vertebrata discovered by Dr. F. V. Hayden, during the expedition to the Sioux country under the command of Lieut. G. K. Warren, P. Acad. Nat. Sci. Phila., 8, 311–312, 1856.
Leidy, J.: Description of Emys jeanesi n. sp., Emys haydeni n. sp., Baena arenosa n. g. n. sp., and Saniwa ensidens n. g. n. sp., P. Acad. Nat. Sci. Phila., 1870, 123–124, 1870.
Leidy, J.: Remarks on fossil vertebrates from Wyoming, Am. J. Sci., 2, 372–373, 1871.
Li, D.-Q., Zhou, C.-F., Li, L., Yang, J.-T., Li, L., and Rabi, M.: The sinemydid turtle Ordosemys from the Lower Cretaceous Mengyin Formation of Shandong, China and its implication for the age of the Luohandong Formation of the Ordos Basin, PeerJ, 7, e6229, https://doi.org/10.7717/peerj.6229, 2019.
Lima, F. C., Santos, A. L. Q., Vieira, L. G., Da Silva-Junior, L. M., Romão, M. F., De Simone, S. B. S., Hirano, L. Q. L., Silva, J. M. M., Montelo, K. M., and Malvásio, A.: Ontogeny of the Shell Bones of Embryos of Podocnemis unifilis (Troschel, 1848) (Testudines, Podocnemididae), Anat. Rec., 294, 621–632, https://doi.org/10.1002/ar.21359, 2011.
Limaverde, S., Pêgas, R. V., Damasceno, R., Villa, C., Oliveira, G., Bonde, N., and Leal, M. E. C.: Interpreting character variation in turtles: Araripemys barretoi (Pleurodira: Pelomedusoides) from the Araripe Basin, Early Cretaceous of Northeastern Brazil, PeerJ Preprints, 6, e27262v1, https://doi.org/10.7717/peerj.9840, 2018.
Lydekker, R.: On certain chelonian remains from the Wealden and Purbeck, J. Geol. Soc., 45, 511–518, https://doi.org/10.1144/GSL.JGS.1889.045.01-04.34, 1889a.
Lydekker, R.: Catalogue of the fossil Reptilia and Amphibia in the British Museum (Natural History) Part III. The Order Chelonia, British Museum (Natural History), London, 239 pp., 1889b.
Milner, A. R.: The turtles of the Purbeck Limestone Group of Dorset, southern England, Palaeontology, 47, 1441–1467, https://doi.org/10.1111/j.0031-0239.2004.00418.x, 2004.
Mitteroecker, P., Gunz, P., Windhager, S., and Schaefer, K.: A brief review of shape, form, and allometry in geometric morphometrics, with applications to human facial morphology, Hystrix, 24, 59–66, https://doi.org/10.4404/hystrix-24.1-6369, 2013.
Mullin, S. K. and Taylor, P. J.: The effects of parallax on geometric morphometric data, Comput. Biol. Med., 32, 455–464, https://doi.org/10.1016/S0010-4825(02)00037-9, 2002.
Murta-Fonseca, R. A. and Fernandes, D. S.: The skull of Hydrodynastes gigas (Duméril, Bibron & Duméril, 1854) (Serpentes: Dipsadidae) as a model of snake ontogenetic allometry inferred by geometric morphometrics, Zoomorphology, 135, 233–241, https://doi.org/10.1007/s00435-015-0297-0, 2016.
Myers, E. M., Tucker, J. K., and Chandler, C. H.: Experimental analysis of body size and shape during critical life-history events of hatchling slider turtles, Trachemys scripta elegans, Funct. Ecol., 21, 1106–1114, https://doi.org/10.1111/j.1365-2435.2007.01337.x, 2007.
Pate, J. H. and Salmon, M.: Ontogenetic niches and the development of body shape in juvenile sea turtles, Chelonian Conserv. Bi., 16, 185–193, https://doi.org/10.2744/CCB-1274.1, 2017.
Paterson, J. E., Steinberg, B. D., and Litzgus, J. D.: Effects of body size, habitat selection and exposure on hatchling turtle survival, J. Zool., 294, 278–285, https://doi.org/10.1111/jzo.12176, 2014.
Pérez-García, A.: The European Early Cretaceous Cryptodiran Turtle Chitracephalus dumonii and the Diversity of a Poorly Known Lineage of Turtles, Acta Palaeontol. Pol., 57, 575–588, https://doi.org/10.4202/app.2011.0065, 2012.
Pérez-García, A.: Revision of the poorly known Dorsetochelys typocardium, a relatively abundant pleurosternid turtle (Paracryptodira) in the Early Cretaceous of Europe, Cretaceous Res., 49, 152–162, https://doi.org/10.1016/j.cretres.2014.02.015, 2014.
Pérez-García, A.: The African Aptian Francemys gadoufaouaensis gen. et sp. nov.: new data on the early diversification of Pelomedusoides (Testudines, Pleurodira) in northern Gondwana, Cretaceous Res., 102, 112–126, https://doi.org/10.1016/j.cretres.2019.06.003, 2019.
Pérez-García, A.: A European Cenozoic “Macrobaenid:” New Data about the Paleocene Arrival of Several Turtle Lineages to Europe, J. Vertebr. Paleontol., 40, e1795874, https://doi.org/10.1080/02724634.2020.1795874, 2020a.
Pérez-García, A.: Surviving the Cretaceous-Paleogene mass extinction event: A terrestrial stem turtle in the Cenozoic of Laurasia, Sci. Rep.-UK, 10, 1489, https://doi.org/10.1038/s41598-020-58511-8, 2020b.
Pérez-García, A. and Ortega, F.: Selenemys lusitanica, gen. et sp. nov., a new pleurosternid turtle (Testudines: Paracryptodira) from the Upper Jurassic of Portugal, J. Vertebr. Paleontol., 31, 60–69, https://doi.org/10.1080/02724634.2011.540054, 2011.
Pérez-García, A., Royo-Torres, R., and Cobos, A.: A new European Late Jurassic pleurosternid (Testudines, Paracryptodira) and a new hypothesis of paracryptodiran phylogeny, J. Syst. Palaeontol., 13, 351–369, https://doi.org/10.1080/14772019.2014.911212, 2014.
Pérez-García, A., Espílez, E., Mampel, L., and Alcalá, L.: A new European Albian turtle that extends the known stratigraphic range of the Pleurosternidae (Paracryptodira), Cretaceous Res., 55, 74–83, https://doi.org/10.1016/j.cretres.2015.02.007, 2015.
Pritchard, P. C. H.: Encyclopedia of Turtles. Neptune, TFH Publications, NJ, 895 pp., 1979.
Pritchard, P. C. H.: Evolution and structure of the turtle shell, in: Biology of turtles, edited by: Wyneken J, Godfrey, M. H., and Bels, V., Boca Raton, CRC Press, London and New York, 46–83, 2008.
Procter, J. B.: A study of the remarkable tortoise, Testudo loveridgii Blgr., and the morphogeny of the chelonian carapace, P. Zool. Soc. Lond., 92, 483–526, https://doi.org/10.1111/j.1096-3642.1922.tb02155.x, 1922.
Püntener, C., Anquetin, J., and Billon-Bruyat J.-P.: The comparative osteology of Plesiochelys bigleri n. sp., a new coastal marine turtle from the Late Jurassic of Porrentruy (Switzerland), PeerJ, 5, e3482, https://doi.org/10.7717/peerj.3482, 2017.
R Core Team: R: A language and environment for statistical computing. R Foundation for Statistical Computing, Vienna, Austria, 2020.
Rohlf, F. J.: tpsDig v. 2.16, State University of New York at Stony Brook, New York, 2010.
Rohlf, F. J.: tpsUtility v. 1.52, State University of New York at Stony Brook, New York, 2012.
Rohlf, F. J.: The tps series of software, Hystrix, 26, 9–12, 2015.
Schoch, R. R. and Sues, H.-D.: The origin of the turtle body plan: evidence from fossils and embryos, Palaeontology, 63, 375–393, 2020.
Seeley, H. G.: Index to the fossil remains of Aves, Ornithosauria and Reptilia, from the Secondary system of strata arranged in the Woodwardian Museum of the University of Cambridge, Deighton, Bell and Co., Cambridge, 143 pp., 1869.
Shao, S., Yang, Y., Li, L., Sun, D.-Y., and Zhou, C.-F.: The first juvenile specimen of Manchurochelys manchoukuoensis from the Early Cretaceous Jehol Biota, PeerJ, 5, e3274, https://doi.org/10.7717/peerj.3274, 2017.
Shao, S., Li, L., Yang, Y., and Zhou, C.-F.: Hyperphalangy in a new sinemydid turtle from the Early Cretaceous Jehol Biota, PeerJ, 6, e5371, https://doi.org/10.7717/peerj.5371, 2018.
Smith, H. F., Hutchison, J. H., Townsend, K. E. B., Adrian, B., and Jager, D.: Morphological variation, phylogenetic relationships, and geographic distribution of the Baenidae (Testudines), based on new specimens from the Uinta Formation (Uinta Basin), Utah (USA), PLoS ONE, 12, e0180574, https://doi.org/10.1371/journal.pone.0180574, 2017.
Snover, M. L.: Growth and ontogeny of sea turtles using skeletochronology: methods, validation, and application to conservation, PhD dissertation, Duke University, Durham, North Carolina, 144 pp., 2002.
Somers, A. B., Bridle, K. A., Herman, D. W., and Nelson, A. B.: The restoration and management of small wetlands of the mountains and piedmont in the Southeast: A manual emphasizing endangered and threatened species habitat with a focus on bog turtles, Natural Resources Conservation Service, Greensboro, 2000.
Sweetman, S. C., Martill, D. M., and Smith, G.: Notes on the discovery of two eutherian mammals in the “Mammal Bed” of the Purbeck Group (Early Cretaceous, Berriasian) exposed in Durlston Bay, Dorset, UK, Proceedings of the Dorset Natural History and Archaeological Society, 139, 105–114, 2018.
Szczygielski, T., Slowiak, J., and Dróżdż, D.: Shell variability in the stem turtles Proterochersis spp., PeerJ, 6, e6134, https://doi.org/10.7717/peerj.6134, 2018.
Tong, H., Ji, S.-A., and Ji, Q.: Ordosemys (Testudines: Cryptodira) from the Yixian Formation of Liaoning Province, Northeastern China: New Specimens and Systematic Revision, Am. Mus. Novit., 3438, 1–20, 2004.
Valente, A. L., Cuenca, R., Parga, M. L., Lavín, S., Franch, J., and Marco, I.: Cervical and coelomic radiologic features of the loggerhead sea turtle, Caretta caretta, Can. J. Vet. Res., 70, 285–290, 2006.
Vieira, L. G., Santos, A. L. Q., Moura, L. R., Orpinelli, S. R. T., Pereira, K. F., and Lima, F. C.: Morphology, development and heterochrony of the carapace of Giant Amazon River Turtle Podocnemis expansa (Testudines, Podocnemidae), Pesqui. Vet. Brasil., 36, 436–446, https://doi.org/10.1590/S0100-736X2016000500014, 2016.
West, I. M.: Purbeck Formation – facies and palaeoenvironments, available at: http://www.southampton.ac.uk/~imw/purbfac.htm (last access: 23 April 2021), 2013.
Wickham, H., Chang, W., Henry, L., Lin Pedersen, T., Takahashi, K., Wilke, C., Woo, K., Yutani, H., and Dunnington, D.: ggplot2: Elegant Graphics for Data Analysis, Springer-Verlag, New York, 2021.
Wright, J. L., Unwin, D. M., Lockley, M. G., and Rainforth, E. C.: Pterosaur tracks from the Purbeck Limestone Formation of Dorset, England, P. Geologists' Assoc., 108, 39–48, https://doi.org/10.1016/S0016-7878(97)80004-1, 1997.
Zangerl, R.: The homology of the shell elements in turtles, J. Morphol., 65, 383–406, https://doi.org/10.1002/jmor.1050650302, 1939.
Zangerl, R.: The vertebrate fauna of the Selma Formation of Alabama. Part IV. The turtles of the family Toxochelyidae. Fieldiana, Geology Memoirs, 3, 137–277, 1953.
Zelditch, M. L., Swiderski, D. L., and Sheets, H. D.: A Practical Companion to Geometric Morphometrics for Biologists: Running analyses in freely-available software, Academic Press, 488 pp., 2012.