the Creative Commons Attribution 4.0 License.
the Creative Commons Attribution 4.0 License.
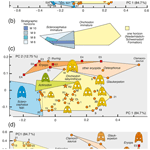
The life cycle in late Paleozoic eryopid temnospondyls: developmental variation, plasticity and phylogeny
Rainer R. Schoch
Eryopid temnospondyls were large apex predators in Carboniferous and Permian stream and lake habitats. The eryopid life cycle is exemplified by Onchiodon labyrinthicus from Niederhäslich (Saxony, Germany), which is represented by numerous size classes from small larvae to heavily ossified adults. Morphometric and principal component analyses provide new insights into ontogenetic changes in O. labyrinthicus, and comparison with adults of other eryopids documents phylogenetic patterns in the occupation of morphospace.
Compared with small specimens of Sclerocephalus spp., immature O. labyrinthicus occupies a neighboring but much larger space, corresponding to a broader range of variation. Adults of Actinodon frossardi map with some juveniles of O. labyrinthicus, whereas other juveniles of the latter lie close to adults of O. thuringiensis, Glaukerpeton avinoffi and Osteophorus roemeri.
Morphospace occupation of adult eryopids is partly consistent with cladistic tree topology, which gives the following branching pattern: Actinodon frossardi forms the basalmost eryopid, followed by Osteophorus roemeri, Glaukerpeton avinoffi and the genus Onchiodon (O. labyrinthicus + O. thuringiensis); then Clamorosaurus nocturnus; and finally the monophyletic genus Eryops. The presumably juvenile skull of Eryops anatinus falls well outside the domains of both adult eryopids and immature O. labyrinthicus, showing a unique combination of juvenile and adult features. Instead, Onchiodon langenhani and the Ruprechtice specimens referred to O. labyrinthicus map within the domain of immature O. labyrinthicus.
Raised levels of variation in O. labyrinthicus coincide with evidence of a stressed habitat, in which limiting factors were fluctuating salinity, absence of fishes, enhanced competition and seasonal algal blooms. The documented broad variation was possibly caused by developmental plasticity responding to fluctuations in lake hydrology and nutrients in this small, short-lived water body.
In extant amphibians, metamorphosis is a drastic transformation in morphology that coincides with a change from an aquatic to a terrestrial existence. In the putative stem group of lissamphibians, the temnospondyls, a drastic metamorphosis has been identified only in a few taxa within the dissorophoid and zatracheid temnospondyls (Werneburg, 1991; Schoch and Fröbisch, 2006; Witzmann and Schoch, 2006). In more basal taxa, such as eryopiform temnospondyls, aquatic gill-bearing larvae also transformed into terrestrial morphs (Witzmann, 2004, 2005), but this change was slow and gradual, with much less specialized larvae transforming slowly into adults (Boy, 1974; Schoch, 2009). Among the latter group, a more enigmatic clade is the eryopids – large apex predators of the late Paleozoic that were preserved in stream and lake deposits and that have often been presumed to have inhabited dry land. The classical taxon, Eryops megacephalus, had a particularly robust skeleton and reached up to 2.5 m length (Sawin, 1941). Similar and related forms were present in France (Actinodon frossardi), Germany (Onchiodon labyrinthicus), Poland (Osteophorus roemeri) and Russia (Clamorosaurus nocturnus). Most of these taxa are known from adults only, with the exception of Onchiodon labyrinthicus, of which a vast growth series exists (Boy, 1990; Witzmann, 2005). It might be expected that metamorphosis, or the ontogenetic transition from water to land, should have left clear-cut traces in the eryopid skeleton. Instead, Boy (1990) and Witzmann (2005) found only gradual changes that must have occurred over a long time interval, similarly to other eryopiforms.
However, Boy (1990) highlighted an unusual extent of variability in the morphology and development of this taxon, with some specimens resembling different developmental stages of other taxa. These potential heterochronies, along with the more general topic of the life cycle in the Eryopidae, form the starting point of the present study. Despite much valuable morphological work (Boy, 1990; Witzmann, 2005; Werneburg, 2008; Werneburg and Berman, 2012), the Eryopidae has not been well studied from a phylogenetic perspective. Establishing a phylogenetic framework is a necessary step towards integrating developmental data in an evolutionary scenario of eryopids.
The present study therefore seeks to integrate the available data (Appendix B) on life cycles in eryopids with patterns of phylogeny. This necessarily starts with the ontogeny of O. labyrinthicus, the ontogenetically best studied and most completely preserved taxon of the clade (Boy, 1990). This taxon is based on rich material preserved with numerous size classes at a single locality and horizon (Niederhäslich near Dresden, Germany), which were first studied by Geinitz (1862) and Credner (1882).
The following objectives are envisioned in the present study: (1) a review of the evidence on morphological changes in O. labyrinthicus with emphasis on newly identified features in the skull, (2) a morphometric analysis of variation and ontogeny in O. labyrinthicus as compared with other eryopiforms, (3) a cladistic analysis of all reasonably preserved eryopids, and (4) an integrated analysis of the evolution of the life cycle in eryopids.
2.1 Material examined
In the present study, 68 specimens of Onchiodon labyrinthicus were examined: MB.Am54, LFUG 13021, LFUG 13047, LFUG 13073, LFUG 13152, LFUG 13292, LFUG 13332, LFUG 13334, LFUG 13337, LFUG 13343, LFUG 13344, LFUG 13353, LFUG 13354, LFUG 13361, LFUG 13373, LFUG 13377, LFUG 13384, LFUG 13388, LFUG 13391, LFUG 13394, LFUG 13398, LFUG 13399, LFUG 13405, LFUG 13418, LFUG 13435, LFUG 13449, LFUG 13459, LFUG 13460, LFUG 13464, LFUG 13469, LFUG 13472, LFUG 13473, LFUG 13501, LFUG 13503, LFUG 13514, LFUG 13516, LFUG 13517, LFUG 13518, LFUG 13529, LFUG 13530, LFUG 13537 (+ LFUG 13538), LFUG 13552, LFUG 13553, LFUG 13554, LFUG 13570, LFUG 13572, LFUG 13609, LFUG 14098, LFUG 14760, LFUG 14830, LFUG 15354, MMG SaP 174, MMG SaP 237, MMG SaP 257, MMG SaP 356, MMG SaP 377, MMG SaP 382, MMG SaP 390, MMG SaP 396, MMG SaP 398, MMG SaP 403, MMG SaP 541, MMG SaP 668, MMG SaP 702, MMG SaP 741, MMG SaP 745, MMG SaP 2172, MMG SaP 2225 and MMG SaP 2228.
2.2 Phylogenetic analysis
2.2.1 Data matrix
The data matrix contains 72 characters from all parts of the skeleton and is based on a matrix first published by Schoch and Witzmann (2009b), to which 17 new characters were added (see Appendix A). Ten additional taxa were included: Sclerocephalus stambergi (Klembara and Steyer, 2012), Sclerocephalus concordiae (from Lake Concordia deposit, St Wendel, Quirnbach Formation; see Schoch and Sobral, 2021), S. bavaricus (Boy, 1988), Osteophorus roemeri (Meyer, 1860), Onchiodon langenhani (Werneburg, 1989), O. thuringiensis (Werneburg, 2008), Glaukerpeton avinoffi (Romer, 1952), Clamorosaurus nocturnus (Gubin, 1983), Eryops anatinus (Broom, 1913) and Eryops sp. (Moran Formation). Two very incompletely known taxa, Onchiodon manebachensis (Werneburg, 1996) and Eryops grandis (Marsh, 1878), have been omitted.
2.2.2 Analysis
The analysis of 72 characters and 25 taxa was conducted in the ACCTRAN mode, under the New Technology search option (Ratchet mode, 1000 replicates). It found four most parsimonious trees requiring 128 steps (CI = 0.609; RI = 0.86). A variant analysis that excluded O. langenhani resulted in a better resolution and three MPTs (127 steps).
2.3 Morphometric analyses
2.3.1 Principal components
The principal component analysis (PCA) employed the software package PAST 4.01 (Hammer et al., 2001). Four ratios, based on six measurements, form the basis of the PCA covariance matrix (Table 2): (a) snout length skull length (SNL SL), (b) interorbital width skull length (IOW SL), (c) postorbital skull width postorbital skull length (POSW POSL) and (d) jugal width skull length (JUW SL). The measurements were not transformed. A total of 68 specimens was analyzed (see Appendix B), which fall into the following taxonomic groups: Onchiodon labyrinthicus (23 specimens of all size classes), O. thuringiensis (1), O. langenhani (1), O. sp. from Ruprechtice (2), Osteophorus roemeri (1), Actinodon frossardi (5), Eryops megacephalus (5), E. anatinus (1), E. sp. from the Moran Formation (1), Clamorosaurus nocturnus (1), Glaukerpeton avinoffi (1), Sclerocephalus haeuseri (13), S. nobilis (12), S. bavaricus (1) and S. concordiae (1). Of these, both O. labyrinthicus and S. nobilis form growth series from one locality and horizon each, whereas S. haeuseri is represented by specimens from different localities and horizons (Fig. 7b). The results of the PCA analysis are listed in Tables 1–2, and morphospace occupation is depicted in Fig. 7, using the first two principal component (PC) axes, which together explain 97.464 % of variance.
2.3.2 Morphometrics
Morphometric ratios were also calculated with PAST 4.01 (Hammer et al., 2001) and are depicted in Fig. 8.
2.4 Abbreviations
2.4.1 Anatomical
Anatomical abbreviations are as follows: ch, choana; d, dentary; df, denticle field; e, ectopterygoid; f, frontal; icl, interclavicle; ju, jugal; la, lacrimal; m, maxilla; n, nasal; p, parietal; pf, postfrontal; pl, palatine; pm, premaxilla; po, postorbital; pp, postparietal; prf, prefrontal; ps, parasphenoid; pt, pterygoid; qj, quadratojugal; sq, squamosal; st, supratemporal; t, tabular; and v, vomer.
2.4.2 Institutional
Institutional abbreviations are as follows: MB, Leibniz-Institut Museum für Naturkunde, Berlin; LFUG, Landesamt für Umwelt, Landwirtschaft und Geologie, Freiberg; MMG, Museum für Mineralogie und Geologie, Dresden; and SMNS, Staatliches Museum für Naturkunde, Stuttgart.
-
Temnospondyli Zittel, 1888
-
Rhachitomi Watson, 1919, sensu Schoch, 2013
-
Eryopiformes Schoch, 2013
-
Eryopidae Cope, 1882
-
Onchiodon Geinitz, 1862
Diagnosis
An eryopid with the following autapomorphy: ectopterygoid fangs reduced in size, much smaller than those of palatine and vomer (Werneburg, 2008).
-
Onchiodon labyrinthicus Geinitz, 1862
Figs. 1–5
Holotype
Large tooth figured by Geinitz (1862, pl. 9, fig. 2).
Type locality and age
Niederhäslich at Freital near Dresden, Saxony, Germany.
Niederhäslich–Schweinsdorf Formation, Lower Rotliegend, Autunian, ? Asselian (lowermost Permian).
Referred material
Altogether, 82 specimens from the type locality and horizon were referred to O. labyrinthicus by Boy (1990). Here, I focus on the material with the best-preserved skulls, which permit focused morphological studies as well as morphometric measurements (see Appendix B). A complete list of studied specimens is provided in the “Materials and methods” section.
Diagnosis
An eryopid growing to 280 mm skull length. Autapomorphies are as follows: (1) skull outline forming wide parabola; (2) preorbital region not longer than twice the skull table; (3) ectopterygoid tusks small; (4) interclavicle long rhomboidal in juveniles, proportionately foreshortened in adults (updated from Schoch and Milner, 2014).
4.1 Historical sketch
In the rich sample from Niederhäslich, Credner (1882) distinguished two size ranges and referred them to separate taxa: immature specimens (larvae and juveniles) were defined as Pelosaurus laticeps and larger juveniles and adults as Onchiodon labyrinthicus. This was an opposite approach to Romer's (1939) placement of all larval temnospondyls from the late Paleozoic deposits within eryopiforms. After Boy (1972) had worked out the distinctive features to separate dissorophoid larvae (Branchiosauridae and Micromelerpetidae) from eryopiforms, he moved on to describe the true larvae and juveniles of selected taxa, including Sclerocephalus haeuseri, Acanthostomatops vorax, Onchiodon labyrinthicus and Glanochthon latirostris (Boy, 1988, 1989, 1990, 1993). These studies provided a wealth of data particularly on the skull and paved the way for a more profound understanding of temnospondyl ontogeny (Boy, 1974; Schoch, 2009).
Boy (1990) and Witzmann (2005) provided many details on the ontogeny of O. labyrinthicus, making this taxon one of the best-studied temnospondyls, and Werneburg (1989, 1993) described related forms from the Thuringian Forest (O. langenhani) and Czech Republic (O. labyrinthicus) although later stressed that the Czech material might pertain to the Polish taxon Osteophorus roemeri (Werneburg, 2008). Many morphological changes have already been reported by Boy (1990), and the development of the hyobranchium and postcranium has been covered in much detail by Witzmann (2005). The emphasis is therefore put on hitherto-unrecognized changes and evidence of variation in the skull, which also remains the most important region for phylogenetic analyses. In Sect. 4.5, changes identified in O. labyrinthicus will be compared with ontogenetic data on other eryopids (O. langenhani and Eryops avinoffi).
4.2 Morphological changes
In the skull, the most conspicuous proportional shifts are the elongation of the preorbital region, the posterior shift of quadrate condyles, the widening of the cheek, and the proportional decrease in orbits and the pineal foramen; all these changes are consistently found in eryopiforms (Boy, 1974; Witzmann, 2006b). More specifically, the following additional changes occur in Onchiodon labyrinthicus (Figs. 1–3).
-
Positive allometry of the nasal is most pronounced in the 10–20 mm range, in which the nasal gains equal length to the frontal.
-
Prefrontal, postfrontal and postorbital initially form slender bars (9.5 mm) but soon expand to become plate-like elements (15–23 mm, Fig. 1). This change is similar in S. haeuseri (Boy, 1988) and Micromelerpeton credneri (Witzmann and Pfretzschner, 2003).
-
The occipital margin of the postparietal and tabular becomes more strongly concave during larval and juvenile growth, but thereafter the trend reverses. Unlike in S. haeuseri, the postparietal lacks posterior lappets (Figs. 1, 2).
-
The lacrimal was highlighted by Boy (1990) as initially bordering the orbit and slowly being separated from the orbit by a medial process of the jugal. This process is present in the smallest larva (9.5 mm) but fails to reach the prefrontal up to a skull length of 30 mm (Fig. 1).
-
The nasolacrimal canal is exceptionally well preserved in O. labyrinthicus and remained open for a longer time than in other temnospondyls (Boy, 1990). In some specimens (25–40 mm range), the canal may split up into two or three branches. As noted by Boy (1990), the canal was closed between a 50 and 60 mm skull length and covered by a low ridge (Figs. 1, 2).
-
The suture between the postorbital and jugal expands in specimens beyond a 40 mm skull length (Fig. 2).
-
The supratemporal is more slender than the parietal up to a 30 mm skull length but thereafter is equal to it or wider (Fig. 1).
-
The pronounced relief on the skull roof was established early, with slightly raised orbit margins taking the lead by a 15 mm skull length, followed by a depression on the lacrimal and a central one on the nasal and then a ridge connecting the orbital margin with the premaxilla by 30 mm and a shorter ridge aligned on the postorbital and lateral supratemporal by 40 mm. Finally, a system of complicated, intersecting ridges appeared on the posterior skull table (Figs. 2–3), a feature shared with the large adult type specimen of O. thuringiensis (Werneburg, 2008).
-
In the palate, the cultriform process is already posteriorly widened in small juveniles and there bears numerous tiny denticles, and the denticle field on the basal plate is emplaced on a large A-shaped platform extending up to the posterolateral corner in small specimens (Fig. 5a).
-
The ectopterygoid tusks are of normal size (compared with other eryopiforms) in juveniles and lag behind the other tusks only in adults; the definitive synapomorphy of Onchiodon therefore developed late, which renders referral of specimens from other localities and horizons to the genus Onchiodon difficult.
-
Boy (1990) studied the change in the dermal ornament in order to distinguish growth stages. He identified an (a) finely pitted larval stage, (b) first generation of (small-scale, low) ridges and (c) second generation of (large-scale, tall) ridges. He emphasized that this sequence paralleled those in Sclerocephalus, Glanochthon and Acanthostomatops. The appearance of tall ridges is also known from Melanerpeton gracile and Micromelerpeton credneri (Werneburg, 1991; Boy, 1995). In the O. labyrinthicus sample, closer examination revealed the following features. The smallest larval specimens have entirely smooth skull bones (9.5 mm) or only very faint pitting (15 mm). The subsequent appearance of ridges started in the posterior skull and squamosal, then expanded onto the jugal (23 mm), and finally completed in the preorbital region (30 mm). As noted by Boy (1990), the development of the ornament in the snout lagged behind the postorbital skull throughout ontogeny, but variation is extreme, as outlined below.
There are no specific, apomorphic larval characters distinguishing O. labyrinthicus from larvae of other taxa. Witzmann (2005) reported ossified ceratobranchials in several larval specimens, and their disappearance coincides with the infolding of dentine and enamel in the teeth and the change in the ornament (stage c), roughly coinciding with Boy's (1990) metamorphosing stage.
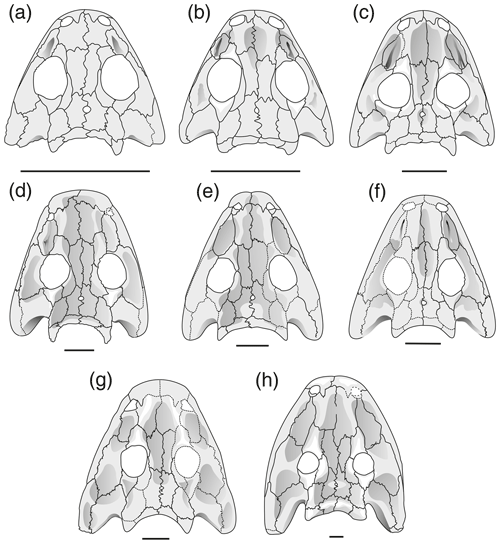
Figure 4Reconstruction of skulls in dorsal view Onchiodon labyrinthicus Geinitz. (a) MMG SaP 237, (b) LFUG 13343, (c) LFUG 13405, (d) MMG SaP 356, (e) LFUG 13391, (f) LFUG 13570, (g) LFUG 13501, (h) LFUG 13292. Darker shading figures depressions on dorsal side of skull roof. Scale equals 10 mm.
4.3 Morphological variation and intraspecific heterochrony
Boy (1990) noticed that the sample of Onchiodon labyrinthicus is particularly variable, which is confirmed by the present analysis. Although there are many polymorphisms, there is no dimorphism apparent in any of the studied traits. For instance, the broad-headed specimen reported by Boy (1990, Fig. 2c) as “forma laticeps” (LFUG 13530, Fig. 1a) is here found to cluster in a wide range of continuous variations instead of being separate from others. Aside from regular cases, there is broad variation in features that are not known to be variable in other taxa, and several traits vary more extremely than known from temnospondyls. As a comparison of the variational range, the eryopiform Sclerocephalus spp. were analyzed. Despite stemming from one locality and horizon, the variation in O. labyrinthicus was found to be greater than in the Sclerocephalus sample, even though the latter is from a wide range of horizons and has been assigned to different species (see “Materials and methods” and Fig. 7).
-
Distance between the nares. The width of the anterior nasal varies greatly but generally decreases in larger specimens relative to skull length.
-
Shape of orbits. This trait ranges from entirely round (length width = 1) to elongate oval (length width = 1.5). Whereas the continuous range is present from larvae to large juveniles, the adult skulls have round orbits.
-
Size of orbits. Boy (1990) emphasized small orbits in his forma laticeps, a trait also found in other specimens throughout the size range. The general negative allometry of orbit size is found to vary broadly in medium-sized specimens, with extreme points formed by “slender-headed morphotype” LFUG 13570 (large orbits) and “Onchiodon type” MMG S356 (small orbits).
-
Interorbital distance. This variation (0.18–0.27) is greater than in other taxa and does not depend on ontogenetic change. The smallest specimens have very similar values to the adults, with broad variation in juveniles (30–45 mm skull length).
-
Posterior skull table (width length). This trait varies broadly in larvae and juveniles (1.7–2.1), contrasting a minor variation in immature Sclerocephalus spp. (1.4–1.65).
-
Interclavicle size. This is the size of the interclavicle compared to skull length (Fig. 5).
-
Interclavicle shape. This is the length width ratio of the interclavicle (see Boy, 1990; Fig. 6 in this work).
-
Ridges. This is the presence and height of ridges on the skull roof (Fig. 2).
-
Occipital margin. The strongly concave occipital margin was established early in ontogeny (by 40 mm skull length) and persisted in most specimens of O. labyrinthicus (Figs. 1–2).
-
Bone thickness. Dermal skull bones as well as endochondral elements in the postcranium vary extremely in thickness. This is most apparent in the 30–60 mm range, thus spanning the late larval, metamorphic and juvenile stages of Boy (1990). Correlation of bone thickness with other traits is analyzed in the next section (Sect. 4.3).
-
Ornament. The variation in the ornament is also considerable but does not strictly correlate with bone thickness. Even in large juveniles (45–60 mm skull length), the ornament varies between poorly ornamented (LFUG 13501, finely striated snout and faint stage-b ornament in the postorbital skull) and fully established tall ridges (MMG SaP 356, extreme version of stage c throughout skull); the large skull LFUG 13570 is intermediate, with a faintly ornamented snout and tall ridges in some places on the frontals and postorbital table. In the 30–45 mm range, the variants are numerous, including the size of the polygons, height of ridges and extent of regional differentiation.
Some morphological variation was caused by dissociated variation in development, such as the extension of the lacrimal towards the orbit and naris, the positive allometry of the nasal, the width of the jugal, and the dermal ornament. In these features, the pace at which changes occurred differed between specimens, resulting in infraspecific heterochronies as described by Reilly et al. (1997). For instance, features in some specimens that developed at a reduced rate compared to conspecifics are referred to as pedotypic and those with a higher rate as peratypic, as contrasted with pedomorphosis and peramorphosis in the comparison between species. In O. labyrinthicus, both pedotypy and peratypy are abundant, probably produced by accelerated and decelerated developmental rates, respectively. These traits will be analyzed further in the next section.
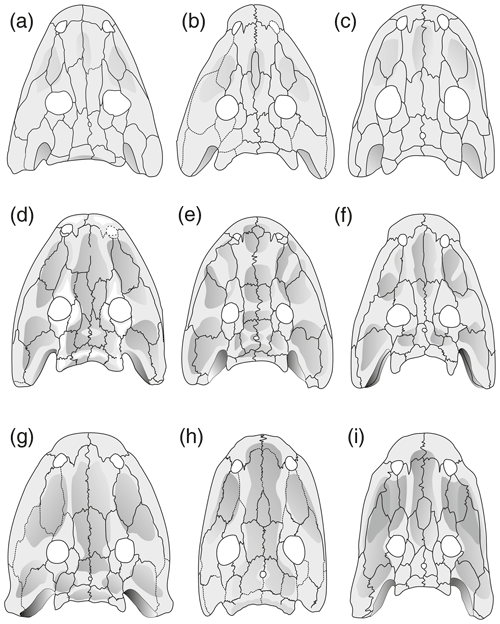
Figure 6Cranial morphology in eryopid temnospondyls, exemplified by reconstructed skull dorsal views. (a) Actinodon frossardi (after Werneburg, 1997), (b) Osteophorus roemeri (after Meyer, 1860), (c) Glaukerpeton avinoffi (after Werneburg and Berman, 2012), (d) Onchiodon labyrinthicus (after Boy, 1990), (e) Onchiodon thuringiensis (after Werneburg, 2008), (f) Clamorosaurus nocturnus (after Gubin, 1983, and photographs courteously provided by Ralf Werneburg), (g) Eryops sp. from the Moran Formation (MCZ 1914), (h) Eryops anatinus (AMNH 4310), (i) Eryops megacephalus (MCZ 1129). Darker shading figures depressions on the dorsal side of the skull roof.
4.4 Morphometric analysis of ontogeny and variation
The key measurements analyzed here are (a) proportion of posterior skull table (width length), (b) length of preorbital region skull length, (c) interorbital distance skull length and (d) jugal width skull length. Of these, a is known to decrease and b and d are known to increase with ontogeny in many temnospondyls, whereas c often changes with development but in different directions in different clades (Bystrow and Efremov, 1940; Boy, 1972; Witzmann and Pfretzschner, 2003; Witzmann, 2006b). Characters a–d were studied in a principal component analysis, as detailed in the “Materials and methods” section. The following patterns were identified.
Larvae of Onchiodon labyrinthicus vary broadly in PC1–PC2, mostly in the proportion of the posterior skull but also in the length of the snout.
In PC1–PC3, larvae of O. labyrinthicus and Sclerocephalus spp. have extreme positive PC3 values, adults of eryopids instead have low positive or negative values, and juveniles of O. labyrinthicus occupy a vast domain ranging from low positive to high negative values (Fig. 7a).
Variation in O. labyrinthicus is far greater than in Sclerocephalus, despite the fact that the Onchiodon sample is from a single locality and horizon, whereas the Sclerocephalus sample includes specimens attributed to different stratigraphical levels and species (Fig. 7a).
Some juvenile specimens of O. labyrinthicus resemble A. frossardi and even S. haeuseri in the proportions of the preorbital region and cheek and are here referred to collectively as slender-headed morphotypes. In LFUG 13570, the snout, interorbital distance and jugal are particularly narrow, giving an overall slender skull (Figs. 2a, 3g, 4f). However, these proportions are within the variational range of O. labyrinthicus, as documented by the PC analysis (Fig. 7a).
An important pattern is the consistent early appearance of adult traits in some juveniles of O. labyrinthicus, such as a wide interorbital distance, broadened jugal, rounded orbit and short postorbital skull (MMG SaP 356). In all these features, larval and juvenile specimens of O. labyrinthicus overlap with immature specimens of Sclerocephalus spp. on the one hand and adult Onchiodon spp. on the other (Fig. 7a). Such patterns of a “premature adult morphotype” are unknown in other temnospondyls, notably among the well-studied Sclerocephalus spp., Glanochthon spp., Acanthostomatops vorax and Micromelerpeton credneri, which have been reexamined for the present study.
When additional traits (bone thickness, ornament type) are mapped onto the occupation of morphospace, further patterns emerge.
The premature adult morphotype (Fig. 5d, MMG SaP 356, no. 8 in Fig. 7) has a robust postcranium, prominent tabular horns, a broad snout, a coarse ornament formed by tall ridges and large polygons, and a pronounced relief. Of all specimens in the sample, it maps nearest to the adult of O. labyrinthicus.
A second morph shares a robust postcranium and thick bones but has small polygons and low ridges, a very wide interorbital region, and a slender jugal (LFUG 13391, no. 9 in Fig. 7). This maps closer to other juveniles of O. labyrinthicus, but some traits may still be referred to as premature adult.
The third morph represents the slender-headed morphotype defined above, which has thin bones, a weak postcranium and slender snout and is represented by two specimens (LFUG 13609, no. 5 in Fig. 7c, and LFUG 13570, no. 10 in Fig. 7c).
The thin-boned specimen LFUG 13501 (Fig. 2b, no. 23 in Fig. 7) maps close to the slender-headed morphotype specimens but nearer to the region of the larval specimens. Despite a large size, it combines a poorly developed ornament with a wide jugal and a gracile postcranium.
The forma laticeps of Boy (1990) combines a broad interorbital region with thick dermal bones and a heavy postcranium but is located far away from the premature adult morphotypes, close to the region of Sclerocephalus spp.
Features that resemble immature ancestral states (e.g., slender-headed morphotype) fall within the pedotypic pattern of Reilly et al. (1997), whereas the characteristic traits of the premature adult morphotype exemplify peratypy. The above examples show that there is no clear-cut dimorphism in which the studied traits are either peratypic or pedotypic. Instead, each specimen represents a unique combination of traits. The early formation of robust postcrania is certainly peratypic but does not always co-occur with an adult cranial morphology. Likewise, the slender-skulled morphs may have either thick or thin dermal bones and robust or weak postcrania. Developmental rates of different traits were clearly dissociated.
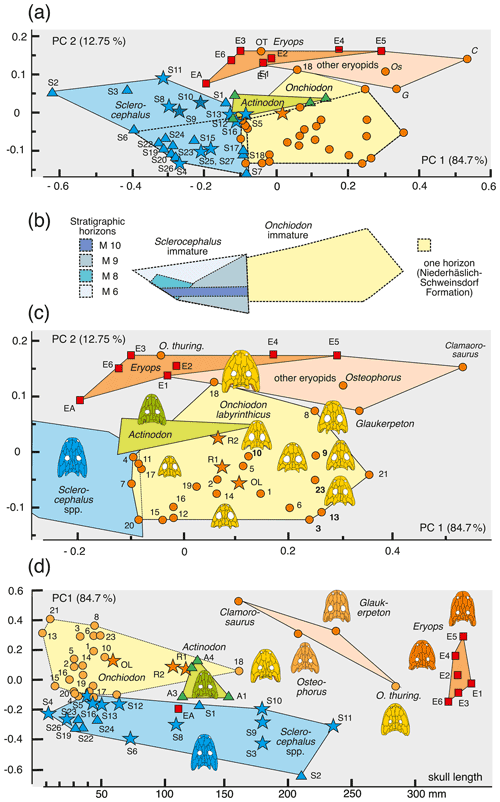
Figure 7Morphospace occupation of eryopiform skulls, showing differences in ontogenetic change and morphometric variance between Onchiodon labyrinthicus and Sclerocephalus spp. and adult skulls of other eryopids. (a) PC1–PC2 axes, (b) areas occupied by immature Onchiodon and Sclerocephalus compared, (c) close-up of (a) with focus on variation in O. labyrinthicus, and (d) PC1 plotted against size.
4.5 Ontogeny and phylogeny in morphospace
When additional taxa are added to the PC analysis, the ontogenetic changes reported in Sect. 4.4 can be put into a phylogenetic perspective. To this end, adults of other eryopid taxa plus specimens representing the major ontogenetic stages of Sclerocephalus haeuseri were added, which gave the following results.
Larvae of Onchiodon labyrinthicus and Sclerocephalus haeuseri occupy partially overlapping or neighboring regions in PC1–PC2 and PC1–PC3. In these spaces, they map in between adult S. haeuseri and small O. labyrinthicus, based largely on the more slender and elongate posterior skull table in S. haeuseri.
Sclerocephalus haeuseri samples from three different horizons in the Saar-Nahe basin were added, namely the Jeckenbach (M6), Klauswald (M9K) and Pfarrwald (M9P) localities (Schoch and Witzmann, 2009a). Adult S. haeuseri maps well outside the domain of adult eryopids and O. labyrinthicus in PC1–PC2 and PC1–PC3, where they form a single cluster. This is contrasted by PC2–PC4, where classical S. haeuseri (Pfarrwald locality) is far apart from the Jeckenbach and Klauswald samples.
Adult Actinodon frossardi maps within a cluster of juvenile O. labyrinthicus, well apart from adult Eryopidae and close to the region of adult Sclerocephalus spp. In both the PC1–PC2 and PC1–PC3 spaces, the type skull of A. frossardi (MNHN 1908-20-6, Muse Formation; Werneburg and Steyer, 1999) is closer to the Sclerocephalus cluster than the Dresden specimen of A. frossardi (MMG FrP1, Millery Formation; Werneburg, 1997). As apparent in PC1–PC2 and PC1–PC3, A. frossardi and eryopids share similar proportions of the posterior skull table, which corroborates the phylogenetic placement of this taxon with the Eryopidae (Werneburg, 1997; Werneburg and Steyer, 1999).
Onchiodon langenhani falls within immature O. labyrinthicus in PC1–PC2 and PC4–PC2 but is clearly outside that cluster in PC1–PC3, where it groups with adult eryopids instead. This taxon may be a close relative of either O. labyrinthicus or O. thuringiensis (being roughly coeval with the latter), but as adults remain unknown from the type locality, the placement is difficult. At any rate, the PC analysis is consistent with the hypothesis that O. langenhani may be referred to the genus Onchiodon.
Osteophorus roemeri (Meyer, 1860), based on a single lost specimen from Lwówek Śląski (SW Poland), has been referred to Eryopidae (Boy, 1990) and suggested to be conspecific with material from Ruprechtice (Liberec, Czech Republic) by Werneburg (2008). In PC1–PC2 space, the two Ruprechtice skulls (R1, R2) map with juvenile O. labyrinthicus and O. langenhani, whereas Osteophorus roemeri clusters with adult eryopids, close to Glaukerpeton avinoffi. This is consistent with the size difference in the Ruprechtice and Lwówek Śląski material, as the latter was reported to have had a 207 mm skull length.
Glaukerpeton avinoffi (Romer, 1952) groups in a domain with Eryops spp. and O. thuringiensis in the PC space, albeit at a distance from both adult Eryops spp. and Onchiodon spp. This confirms the phylogenetic hypothesis of Werneburg and Berman (2012), in which Onchiodon and Eryops form sister taxa and Glaukerpeton is their closest relative.
Eryops anatinus (AMNH 4310), a taxon based on a single, relatively small skull (130 mm), maps well outside the adult eryopid cluster, closer to Sclerocephalus spp. than to A. frossardi or immature O. labyrinthicus. In the case that E. anatinus represents an immature E. megacephalus, the ontogeny of that taxon would differ substantially from that of O. labyrinthicus. Alternatively, E. anatinus may be interpreted as a small adult of a dwarf Eryops species, which is supported by its heavy ossification, general adult proportions (preorbital region, jugal width, pronounced relief) and the outline of the orbits with strongly convex lateral emarginations resembling the largest specimens of S. haeuseri (Schoch and Witzmann, 2009b).
The type species E. megacephalus from the early Permian of Texas is present in the Putnam, Admiral, Belle Plains, Clyde and Arroyo formations, spanning most of the Asselian–Kungurian interval (Schoch and Milner, 2014). Specimens of E. megacephalus (AMNH 4189) and E. sp. from the Moran Formation (MCZ 1914) are closely located in the PC1–PC2 and PC1–PC3 spaces.
Clamorosaurus nocturnus and C. borealis (Gubin, 1983), from the late Permian (Ufimian) of Russia, are the stratigraphically youngest eryopids, characterized by an extremely abbreviated posterior skull table and a snout region similar to that of E. megacephalus, with a stepped lateral margin and a proportionately very long snout. The more completely known C. nocturnus was included here and maps with adult eryopids in PC1–PC2 but far apart from all other specimens in PC1–PC3, due to its small interorbital distance.
The interorbital distance appears isometric at first sight in both O. labyrinthicus (0.16–0.27) and S. haeuseri (0.16–0.2), with the two taxa falling within neighboring, slightly overlapping domains. However, the large specimens of O. labyrinthicus range near the extreme juvenile values, whereas the bulk of the juveniles are in the 0.19–0.22 range, which suggests an increase in interorbital distance with age. In S. haeuseri, this is only the case in some populations (Schoch and Witzmann, 2009a).
The posterior skull table decreases gently in width in some populations of S. haeuseri, especially at the type locality Heimkirchen (Boy, 1988). In that species, larvae and juveniles (1.5–1.7) differ only a little from adults (1.8–1.15), with the phylogenetically older S. bavaricus and S. concordiae having the highest values (1.49–1.55). In O. labyrinthicus, larvae and juveniles (1.7–2.1) differ only slightly from adults (1.8), with O. thuringiensis resembling juveniles (1.71) but O. langenhani and the Ruprechtice specimens precisely consistent with adult O. labyrinthicus (1.81–1.83). Osteophorus roemeri (2.05) is much closer to Glaukerpeton avinoffi (2.08) than to any Onchiodon specimen. This result indicates that O. roemeri is unlikely to be conspecific or closely related to the Ruprechtice specimens, which in turn may be closely related to the two Onchiodon species. Adults of Actinodon frossardi overlap in range with O. labyrinthicus and are nearing the range of the ancient Sclerocephalus species.
The details of the cladistic analysis are provided in the “Materials and methods” section.
5.1 Results
The obtained topology encompasses a monophyletic Eryopiformes, composed of the sister taxa Eryopidae and Stereospondylomorpha with dissorophoids and zatracheids nesting below eryopiforms. Stereospondylomorpha are here found to include “Sclerocephalus” stambergi, Sclerocephalus bavaricus, S. concordiae and S. haeuseri as a basal grade or clade, followed by Glanochthon angusta and G. latirostris, with Archegosaurus decheni (+ Platyoposaurus stuckenbergi + Australerpeton cosgriffi) as crown taxa. In turn, Eryopidae includes the successive sister taxa Actinodon frossardi, Osteophorus roemeri, Glaukerpeton avinoffi, Onchiodon labyrinthicus + O. thuringiensis, Clamorosaurus nocturnus, Eryops sp. Moran, E. anatinus and E. megacephalus (Fig. 8). This result was obtained under the exclusion of the poorly known Onchiodon langenhani; upon its inclusion, it nests one node above G. avinoffi but below Onchiodon proper. This is the first time the taxa Osteophorus roemeri and Clamorosaurus nocturnus have been considered, and their positions are stable within the framework.
5.2 Evolutionary history of the Eryopidae
The phylogenetic topology reported here (Fig. 8) confirms Werneburg (1997) and Werneburg and Steyer (1999), who already found the French taxon Actinodon frossardi to nest with eryopids, and the hypothesis of Werneburg and Berman (2012) that Glaukerpeton avinoffi forms the sister taxon of a clade containing Onchiodon and Eryops. Morphospace occupation of A. frossardi agrees with the cladistic analysis in that this taxon is intermediate between Sclerocephalus spp. and other Eryopidae. However, in morphospace (PC1–PC2), G. avinoffi and O. labyrinthicus form the taxa closest to A. frossardi, whereas Osteophorus roemeri is somewhat more distant (Fig. 7).
The obtained framework of relationships further indicates that the genus Onchiodon in its current, wide definition forms a paraphyletic grade towards Clamorosaurus and Eryops. The referral of a taxon as basal as A. frossardi to Onchiodon (Werneburg, 1997) would require the whole clade Eryopidae to be included in that taxon in order to be consistent with phylogenetic systematics. Considering the morphological range of specimens referred to A. frossardi in the literature, the existence of more than one taxon is probable, but this issue needs thorough revision by workers familiar with the French localities and stratigraphy. The specimens included in the present PC analysis might still pertain to a single taxon, and their position in the morphospace between Sclerocephalus and the more advanced Eryopidae is consistent with their possession of eryopid synapomorphies, such as the rounded choana and lacrimal extension towards the naris, along with symplesiomorphies like the absence of an ectopterygoid tooth row.
Whereas the placement of A. frossardi at the base of eryopids appears to be robust, the position of Osteophorus roemeri is less certain. Despite its possession of eryopid characters, mirrored by its appearance with more advanced eryopids in the PCA, this taxon also shares two features with stereospondylomorphs, which are the suture of nasal and maxilla anterior to the lacrimal and the slender anterior snout (Fig. 6). The tentative referral of the Ruprechtice material to O. roemeri (Werneburg, 2008) is an interesting suggestion but is hampered by the loss of the holotype and the poor condition of the Czech material. Judging from the PC analysis, the position of the Ruprechtice specimens among juveniles of O. labyrinthicus, with the more mature specimen close to the domain of A. frossardi, suggests either a more immature state of the Ruprechtice material (consistent with its smaller size) or a more basal phylogenetic position than the other Onchiodon species. This highlights a common ambiguity caused by interspecific heterochrony (Steyer, 2000; Wiens et al., 2005).
The Ruprechtice specimens probably form a separate species characterized by a huge interclavicle having 75 % the length of the skull (Werneburg, 1993), contrasting O. langenhani and O. labyrinthicus in which that value reaches only 50 % (Werneburg, 1989). The only temnospondyl with a comparable size of the interclavicle is Sclerothorax hypselonotus (Schoch et al., 2007), which appears to have been an amphibious capitosaur. A referral of the Ruprechtice material to the genus Onchiodon is presently avoided, as the definitive synapomorphy (reduced ectopterygoid tusks) is a feature only present in much larger, fully mature specimens that are not known from Ruprechtice.
In the eryopid lineage, the adult skull underwent a successive broadening, first by increasing the interorbital distance (post-Glaukerpeton taxa) and then by widening the lacrimal, jugal and squamosal. In Onchiodon spp., Clamorosaurus nocturnus and Eryops spp., this reached a peak. In Eryops megacephalus, the tip of the snout was expanded and the lateral premaxilla and anterior maxilla teeth further enlarged. The Permian species of Eryops experienced an elongation of the preorbital region to about 3 times the length of the posterior skull table, which was paralleled by Clamorosaurus nocturnus in Russia.
The lifestyle of eryopids is not as clear as that of other temnospondyls, and it is well possible that lifestyles also differed within the clade. This was suggested by Werneburg and Berman (2012), who reported ossified ceratobranchials in Glaukerpeton avinoffi, which in concert with vestiges of lateral line sulci suggest an aquatic mode of life, highlighting the differences from the supposedly more terrestrial genera Onchiodon and Eryops. Yet the question of how terrestrial any of these taxa were remains undecided, as for instance the dentition of eryopids does not depart much from the pattern of stereospondylomorphs, which were evident fish predators due to their stomach contents and probably spent most of their lives in lakes (Boy, 2003). Much like Sclerocephalus haeuseri, Actinodon frossardi was heavily built and still somewhat more robustly ossified, contrasting the more lightly built genera Glanochthon, Archegosaurus, Korkonerpeton and Cheliderpeton (Schoch and Witzmann, 2009a, b; Werneburg et al., 2020).
Sclerocephalus, Actinodon, Onchiodon and Eryops form a morphocline with ever more robust humeri, heavily ossified vertebrae and an increasing thickness of dermal bones (Werneburg, 2008). Conversely, the less heavy pectoral girdle with a smaller and thinner interclavicle suggests reduced importance to form a heavy anchor for the body under water in adult Onchiodon and Eryops, probably enabling these taxa to walk more frequently over land. This is consistent with the enhanced robustness of the humerus, which is already more differentiated in A. frossardi compared with S. haeuseri (Gaudry, 1867; Meckert, 1993) but substantially more torted with a larger supinator process, deltoid crest, latissimus dorsi process and radial condyle in Glaukerpeton avinoffi (Werneburg and Berman, 2012) and reaches an end point with the massive bone in Eryops megacephalus (Miner, 1925; Pawley and Warren, 2006). Again, this does not imply a fully terrestrial existence for any eryopid, which would also be ecologically questionable given the presence of larger predators in many of the neighboring terrestrial habitats (Romer, 1928).
Despite the mentioned morphological differences, the eryopids were probably fish eaters not unlike the more gracile stereospondylomorphs, and their abundant presence in or around lakes and streams indicates that they were at best amphibious, migrating between water bodies in search of places rich in aquatic prey. The trackways of Limnopus spp. were most likely produced by eryopids migrating between such places. This hypothesis is in line with the slow transformation of larvae into juveniles and adults as found by Boy (1990), by Witzmann (2005) and in the present PC analysis. Like in the genera Sclerocephalus and Glanochthon (Boy, 1988, 1993), there is no evidence of a drastic metamorphic change in O. labyrinthicus, and the consistency in dentition throughout ontogeny suggests that the small ones probably fed on aquatic arthropods, branchiosaurid larvae and baby fishes, whereas the juveniles and adults focused on increasingly larger fishes, such as Paramblypterus with its closed cuirass of relatively thick scales. This has been reported for Sclerocephalus haeuseri which dwelled in larger, deeper lakes that were rich in actinopterygians (Boy, 2003), contrasted by the more gracile genera Glanochthon and Archegosaurus that evidently fed on acanthodians (Boy, 2003). Actinopterygians also form the most likely prey for Onchiodon labyrinthicus and O. thuringiensis, which inhabited small lakes and streams (Werneburg, 2008). The dominance of Eryops spp. in floodplain and stream deposits of the American southwest has long been emphasized (Romer, 1928, 1935; Sander, 1989), and this documents a shift within the eryopid lineage, from (a) inhabitants of larger, deeper water bodies and peat lakes (Actinodon, Osteophorus, Glaukerpeton) not unlike those dwelled in by Sclerocephalus to (b) instable lakes, small ponds, channels and oxbow lakes (Onchiodon) and finally to (c) streams, deltas and floodplains with ephemeral ponds (Eryops). The increasing relief of the skull with its raised orbits adds to this picture, giving a caiman-like fish predator that roamed streams and was more aware of what happened at the shore than deep-water-dwelling, flat-headed stereospondylomorphs that relied more on the lateral sense than on sight.
5.3 The Niederhäslich paleoecosystem
The type locality of O. labyrinthicus, from which the bulk of the analyzed sample stems, forms a rather unique setting (Boy, 1977, 1990; Schneider, 1993). This fossillagerstätte, the lacustrine deposits of Niederhäslich, was interpreted as a set of interconnected water bodies in a 10×6 km large intramontane basin plain (Gebhardt and Schneider, 1993). The richest fauna and classical locality represent a small lake that was seasonally rich in algae and populated by diverse temnospondyl fauna. The lake floor was morphologically differentiated with a seasonally changing shoreline; contemporaneous volcanism plus evaporation had an impact on salinity, and fluctuations in salinity are indicated by thin-shelled bivalves (Schneider, 1993; Reichel, 2006).
In the Niederhäslich lake, fishes were absent, but small invertebrates (ostracods, bivalves) settled in areas with better living conditions on the lake floor (Schneider, 1993). Aquatic tetrapods therefore had to rely on food sources other than the usual fish diet known from other basins. The above-mentioned salinity fluctuations explain the absence of fishes, whereas seasonal algal blooms likely caused increased mortality among the aquatic tetrapods. In addition, it has been concluded that the Niederhäslich lake had a chemocline, separating a freshwater epilimnion from a brine hypolimnion (Gebhardt and Schneider, 1993). Fluctuations of the chemocline form an additional factor likely to have killed the fauna. In combination, this setting formed a particularly stressed environment (Schneider, 1993).
The smallest and most common aquatic tetrapod at Niederhäslich, the branchiosaurid Melanerpeton gracilis, best illustrates the exceptional status of this environment: unlike any other branchiosaurid, this species developed at a fast pace and metamorphosed into a terrestrial morph (Werneburg, 1991; Schoch and Fröbisch, 2006). This indicates that resources were limited by either primary shortage or interspecific competition. Although both explanations find support by the data (e.g., absence of fishes), the competition hypothesis is more likely to have been a general factor, as there were four temnospondyl taxa present in the lake. Compared with other lake deposits in the late Paleozoic Rotliegend sequence of Europe, this is exceptional diversity.
The Niederhäslich lake community was populated by larval and juvenile Melanerpeton, Branchierpeton, Acanthostomatops and Onchiodon, with only Branchierpeton also regularly present with adults. Boy (1989, 1990) and Werneburg (1991) studied the size range and frequency distribution of specimens for these taxa, showing that except for Branchierpeton, adults were only rarely preserved and thus likely died during the mating season.
The abundance of larvae and juveniles means that some or all of these taxa might have competed for food at some stage of their development. That density was high is documented by fossil evidence of cannibalism in Melanerpeton gracile (Witzmann, 2009); cannibalism in extant salamanders has been observed in populations with high density (Degani, 1993). This completes the list of factors that probably shaped the stressed Niederhäslich environment: high density, enhanced competition, limited variety of prey, absence of fishes, hydrological instability and seasonal poisoning (Boy, 1977; Schneider, 1993).
Responding to the resulting selection pressure, Niederhäslich temnospondyls apparently explored two alternative strategies: (1) to grow into an aquatic niche in which to exploit some food source alone (Branchierpeton) or (2) to leave the lake as soon as possible because of general resource restrictions and habitat instability (Melanerpeton, Acanthostomatops, Onchiodon). Branchierpeton appears to have been the only perennibranchiate taxon in that ecosystem (Schoch, 2009). For the putative filter feeder Melanerpeton, the primary food source appears to have been plankton, and the carnivorous phase known from neotenic branchiosaurids was probably skipped (Schoch, 2014a). For Branchierpeton, conchostracans, ostracods and other aquatic arthropods might have been the most attractive food, which was exploited by similar-sized neotenic branchiosaurids in many other lakes (Boy, 2003) but not here, where Melanerpeton evidently left the lake early. Consequently, Branchierpeton was smaller than its relative Micromelerpeton, which focused on branchiosaurid prey instead (Boy, 2003). Melanerpeton and Branchierpeton were probably preyed upon by Onchiodon, whose juveniles were abundant (30–45 mm skull length). Hence, juvenile Onchiodon probably formed the apex predator in the Niederhäslich ecosystem and is likely to have eaten all smaller tetrapods including smaller conspecifics, a pattern not uncommon among Paleozoic temnospondyls (Boy, 1990; Schneider, 1993; Schoch, 2014b). The rarest taxon, immature Acanthostomatops, probably dwelled in slightly different habitats (possibly the shore) and might have used other resources instead.
5.4 Developmental plasticity and pressure for habitat change
The present study identified an enhanced level of variation among immature specimens of O. labyrinthicus at Niederhäslich. This is demonstrated by the occupation of a morphospace area (PC1–PC2) about double the size of a similarly large sample of Sclerocephalus spp. (Fig. 7b). It is also evident from the variational range in the dermal ornament and bone thickness. Moreover, variation in O. labyrinthicus is larger at Niederhäslich than variation in S. spp. was in a range of localities and horizons.
The question emerges of which factor might best explain the pattern of enhanced variation in O. labyrinthicus. As the PC analyses have demonstrated, variation did not result in distinct morphs (e.g., slender-headed morphotype versus premature morphotype without intermediates) but gave a rather continuous range of dissociated features.
An enhanced level of variation may be caused by the following alternative factors: it might (1) be entirely genetic, without a component of plasticity (i.e., polymorphism); (2) represent a blurred pattern of sexual dimorphism; or (3) be a plastic response to fluctuating environmental parameters (i.e., polyphenism).
Among these, the sexual-dimorphism hypothesis is the least plausible for the studied sample of O. labyrinthicus because this sample consists to a large extent of juveniles that were probably not yet sexually mature. Of course, maturity cannot be inferred with certainty from morphology alone, but the documented size and frequency distribution of O. labyrinthicus (Boy, 1990) indicate that sexually mature specimens were preserved only during the breeding season. In this pattern, successive discontinuous clusters of a few larger specimens probably represent mature specimens, whereas the continuous (and highly variable) range of many large juveniles indicates that these growth stages still lived in the lake. Thus, this pattern, along with the continuous variation and the free combination of traits (e.g., bone thickness, ornament, jugal width, interorbital distance, postorbital skull length), is not consistent with any dimorphism hypothesis.
The genetic-polymorphism hypothesis cannot be tested in fossils and therefore may not be ruled out entirely. There is no way to conclude on the likelihood of this factor in any fossil data set, and therefore this alternative remains on the table.
The third alternative explanation of enhanced variation is a plastic response to environmental fluctuations (polyphenism). This is accomplished by a sufficiently broad reaction norm (Schlichting and Pigliucci, 1998). The question remains whether this can be ruled out or made plausible by some additional evidence. Here I argue that in the case of O. labyrinthicus from Niederhäslich, polyphenism may be suggested by (1) parallels between the sample of O. labyrinthicus and the probably plastic populations of branchiosaurids being apparent (see below) and (2) the paleoecological and environmental data presented by Schneider (1993).
In branchiosaurids, the rate of development differed between samples of different lakes and sometimes even between samples from successive layers of the same lake deposit, which has been interpreted as caused by plastic responses to various hydrological conditions (Schoch, 2014a). These examples all stem from stable, relatively deep lakes in the Saar-Nahe basin (Boy, 2003), where plasticity is interpreted as a strategy to settle in a wide range of different habitats. Instead, in the sample of O. labyrinthicus from Niederhäslich, enhanced variation occurred within the same habitat. The lacustrine limestone at Niederhäslich is interpreted as a time-averaged deposit (Boy, 1977), which means that tetrapod skeletons from different seasons may co-occur on closely set bedding planes or may even be found on the same bedding plane. This, in turn, could explain why a broad variation in O. labyrinthicus does not necessarily imply variation within a population of a single season but probably includes specimens that died during successive seasons that were blended together by time averaging. Hence, the broad variation could have been created by seasonal fluctuations in the variational range. If this was the pattern behind the time-averaged variation in O. labyrinthicus at Niederhäslich, plastic responses to fluctuating environmental conditions would be a plausible explanation for the enhanced level of variation. Variation in bone thickness and the dermal ornament would fit with this pattern, as such variation has long been known to be caused by environmental fluctuations in extant lissamphibians (Duellman and Trueb, 1994).
As explored by Michimae (2006), polyphenism emerges from a lucrative strategy in aquatic salamanders. For instance, Hoffman and Pfennig (1999) reported cannibalistic polyphenism in tiger salamanders (Ambystoma tigrinum), where the emergence of cannibalistic morphs is triggered in habitats with high population density. However, a dimorphic pattern has not been found in O. labyrinthicus, and the dentition does not vary significantly within the studied sample.
In the case that the broad variation in O. labyrinthicus was indeed caused by polyphenism, these responses were most likely triggered by hydrological fluctuations. Schneider (1993) suggested that volcanic ashfall raised the salinity of the lake during the short term, whereas subsequently inflowing meteoric water lowered that level in the epilimnion. The resulting fluctuation put the freshwater inhabitants, including tetrapods, under stress. This may have caused a size reduction or higher mortality in ostracods, the documented prey of branchiosaurids. As a consequence of food shortage, earlier metamorphosis (or cannibalism) in Melanerpeton, a reduced growth rate in Branchierpeton and a food shortage with premature transformation in Onchiodon labyrinthicus were possible results. In the latter, the premature adult pattern might have resulted from a plastic response to such stress, marking an early emigration out of the lake habitat. Juveniles resembled the non-lake-dwelling adults, even though they had not yet left the lake. Conversely, the slender-headed morphotype could represent a relaxed response, such as when exploiting the lake for somewhat longer during stable phases with low salinity and larger or more abundant prey. The morphological resemblance to Actinodon and Sclerocephalus would be greatest in specimens that grew up in such a phase. At the same time, the continuous rather than dimorphic variation suggests that salinity and other parameters fluctuated gradually rather than between extremes.
Depending on the availability of food, O. labyrinthicus might have (a) left the lake earlier or later and/or (b) simply developed at a faster (peratypic) or slower (pedotypic) pace. These different triggers may also be responsible for the continuous variation and the polyphenic pattern of morphs overlapping with Actinodon, Sclerocephalus, adult Onchiodon and other eryopid adults, as shown in Fig. 7.
In sum, polyphenism in O. labyrinthicus forms an interesting possibility but requires critical examination of additional data sources, such as paleohistology. Future projects should expand on this problem, which is beyond the scope of the present study.
A1 Character list
1. | Premaxilla (alary process). Absent (0) or present (1). |
2. | Premaxilla (prenarial portion). Short (0) or expanded anteriorly by about the length of the naris (1). |
3. | Premaxilla (outline). Parabolically rounded (0) or box-like, anteriorly blunt (1). |
4. | Snout (internarial distance). Narrower than interorbital distance (0) or wider (1). |
5. | Snout (margin). Straight (0) or laterally constricted at level of naris (1). |
6. | Rostrum. Absent (0) or present (1). |
7. | Internarial fenestra. Absent (0) or present (1). |
8. | Orbits. Round to slightly oval (0) or elongated oval (1). |
9. | Orbits. Ends rounded (0) or pointed (1). |
10. | Maxilla (anterior margin). Straight (0) or laterally convex due to enlarged teeth (1). |
11. | Maxilla (contact to nasal). Absent, separated by lacrimal (0), or present (1). |
12. | Nasal (lateral margin). Straight (0) or stepped, with lateral excursion anterior to prefrontal, accommodating narrower lacrimal (1). |
13. | Lacrimal (length). As long as nasal (0), shorter than nasal (1) or much abbreviated (2). |
14. | Lacrimal (width). Lateral suture parallels medial one (0) or lateral suture posterolaterally expanded to give broader preorbital region (1). |
15. | Preorbital region (length). Less than twice the length of posterior skull table (0) or more (1). |
16. | Prefrontal jugal (contact). Absent (0) or present (1). |
17. | Prefrontal (anterior end). Pointed (0) or wide and blunt (1). |
18. | Frontal nasal (length). Frontal as long or longer than nasal (0) or shorter (1). |
19. | Interorbital distance. Narrower than orbital width (0) or wider (1). |
20. | Lateral line (sulci). Absent in adults (0) or present (1). |
21. | Posterior skull table (length). Length measures 0.6–0.7 times the width (0), 0.7–0.8 (1), larger than 0.8 (2) or 0.4–0.6 times the width (3). |
22. | Intertemporal. Present (0) or absent (1). |
23. | Postorbital. Long triangular, wedged deeply between squamosal and supratemporal (0), or short (1). |
24. | Squamosal embayment (size). Wide, giving semilunar flange on squamosal (0), or slit-like, with thin flange on squamosal (1). |
25. | Tabular (ventral crest). Absent (0) or present (1). |
26. | Jugal (preorbital expansion). Absent in adults (0) or present (1). |
27. | Ornament. Polygons and short ridges (0) long ridges arranged radially (1). |
28. | Vomer. Smooth (0) or with paired depressions anteriorly (1). |
29. | Vomerine tusks. Anterolateral to choana, transverse row (0), or well anterior to choana, sagittal row (1). |
30. | Anterior palatal openings. Absent (0) or present (1). |
31. | Choana (width). Elongated oval or slit-like (0), round (1), or medially expanded (2). |
32. | Premaxilla. Borders choana (0) or does not (1). |
33. | Palatine, ectopterygoid (continuous tooth row). Absent (0) or present (1). |
34. | Palatine. Fangs and no more than three–four extra teeth (0) or five or more extra teeth (1). |
35. | Ectopterygoid (tusks). Present (0) or absent (1). |
36. | Parasphenoid. Denticle field on plate triangular (0) or round (1). |
37. | Basipterygoid ramus (length). Transverse, rod-like (0), or short, without medial extension (1). |
38. | Basicranial articulation. Moveable overlap (0) or tightly sutured (1). |
39. | Carotid foramina (entrance). Anteromedial on basal plate, close to cultriform process (0), or at posterolateral corner of plate (1). |
40. | Vomer. Separated by pterygoid from interpterygoid vacuity (0) or bordering that opening (1). |
41. | Cultriform process (width). Throughout of similar width (0) or posteriorly expanding abruptly to about twice the width (1). |
42. | Stapes (quadrate process). Absent (0) or present (1). |
43. | Interclavicle (adult shape). As wide as long (0) or longer than wide (1). |
44. | Interclavicle (width). As wide as or wider than posterior skull table (0) or narrower (1). |
45. | Interclavicle (size). Shorter than posterior skull table (0) or longer than half of skull length (1). |
46. | Interclavicle (posterior margin). Triangular, pointed (0), or rounded to blunt (1). |
47. | Interclavicle (outline). Rhomboid (0) or quadrangular to pentagonal (1). |
48. | Humerus (entepicondylar foramen). Present (0) or absent (1). |
49. | Humerus (supinator). Present (0) or absent (1). |
50. | Humerus. Short with slow growth rate in larvae (0) or long due to rapid growth (1). |
51. | Femur. Intercondylar fossa on dorsodistal surface forming deep trough (0) or shallow groove (1). |
52. | Pubis. Ossified (0) or unossified (1). |
53. | Ilium. Shaft kinked, posteriorly directed (0), shaft straight and dorsal with broadened end (1), or shaft straight posterodorsally directed (2). Unordered. |
54. | Ribs. Short (0), long and rod-like with small uncinates (1), or long with blade-like uncinates (2). Unordered. |
55. | Interpterygoid vacuities. Longer than vomer and premaxilla (0) or equal to or shorter (1). |
56. | Neurocranium. Cartilaginous or only partially ossified (0) or fully ossified with sphenoid and ethmoid portions (1). |
57. | Squamosal embayment. Framed by parallel squamosal and tabular margins (0) or forming medially rounded extension, constricting the posterior skull table (1). |
58. | Supratemporal. Less than or about 2 times longer than wide (0) or more than 2.5 times longer than wide (1). |
59. | Squamosal. Posterior part as wide as quadratojugal (0) or markedly narrower (1). |
60. | Jugal. Wider than orbit (0) or markedly narrower (1). |
61. | Premaxilla. Lateral margin straight (0) or bulging laterally (1). |
62. | Snout. Shorter than 2 times the length of postorbital skull table (0) or as long or longer (1). |
63. | Lacrimal. At least two-thirds the length of the preorbital skull (0) or shorter (1). |
64. | Quadrate. Wedging in between quadratojugal and squamosal posteriorly (0) or offset from the posterior margin of the dermal cheek bones (1). |
65. | Ridges. Skull roof without (0) or with (1) prominent ridges connecting raised orbits with nares and tabular horn. |
66. | Sphenethmoid. Fully ossified late in ontogeny (0) or before adult stage (1). |
67. | Ectopterygoid. Shorter or equal in length to palatine (0) or longer (1). |
68. | Septomaxilla. With dorsal exposure at posterior margin of naris (0) or without dorsal exposure (1). |
69. | Interfrontonasal. Absent (0) or present (1). |
70. | Infraorbital bar. Narrower than interorbital distance (0) or equal to or wider (1). |
71. | Pterygoid. Without transverse process (0) or with such process (1). |
72. | Ectopterygoid fangs. Similar to palatine fangs (0) or greatly reduced in size (1). |
A2 Character–taxon matrix
Dendrysekos helogenes |
1 0 0 0 0 0 0 0 0 0 0 0 0 0 0 0 0 0 0 0 0 0 0 0 0 0 0 0 0 0 0 0 0 0 0 0 0 0 0 1 0 0 0 0 0 0 0 0 0 ? 0 0 0 0 0 0 0 0 0 1 0 0 0 0 0 0 1 0 0 0 0 |
Balanerpeton woodi |
1 0 0 0 0 0 0 0 0 0 0 0 0 0 0 0 0 0 0 0 0 0 0 0 0 0 0 0 0 0 0 0 0 0 0 0 0 0 0 1 0 0 0 0 0 0 0 0 0 0 0 0 0 0 0 0 0 0 0 1 0 0 0 0 0 0 0 0 0 0 0 |
Cochleosaurus bohemicus |
0 1 0 1 0 0 0 0 0 0 0 0 0 0 0 0 0 1 0 0 0 0 0 0 0 0 0 0 0 0 0 0 0 0 0 0 0 0 0 0 0 0 0 0 0 0 0 0 1 0 0 0 0 0 0 0 0 0 0 0 0 0 0 0 1 0 0 0 0 0 0 |
Micromelerpeton credneri |
1 0 0 0 0 0 1 0 0 0 0 0 0 0 0 0 0 0 0 0 0 1 1 0 0 0 0 0 0 0 0 0 0 0 0 0 0 0 0 1 0 0 0 0 0 1 1 1 1 1 0 0 1 0 1 0 0 0 0 1 0 0 0 0 0 0 0 0 0 0 0 |
Acanthostomatops vorax |
1 1 0 0 0 0 1 0 0 0 0 0 0 0 1 0 0 0 1 0 0 1 1 0 0 0 0 0 0 0 0 0 0 0 0 0 0 0 0 1 0 0 0 0 0 1 1 1 0 1 0 1 2 0 1 0 0 0 0 0 0 0 0 0 0 0 0 0 0 1 0 |
Iberospondylus schultzei |
1 0 0 0 0 0 0 0 0 0 0 0 0 0 1 0 0 0 0 0 0 1 0 0 0 0 0 0 0 0 0 0 0 0 0 0 0 0 ? ? 0 0 ? ? ? ? ? ? ? ? ? ? ? 1 1 0 0 0 0 0 0 0 0 0 0 0 0 0 0 0 0 |
Actinodon frossardi |
1 0 1 1 0 0 0 0 0 0 0 0 0 0 1 1 0 0 1 0 0 1 0 1 0 1 0 1 0 0 1 0 0 0 0 0 0 1 1 1 1 ? 0 0 1 1 0 1 0 ? 1 0 ? 2 0 0 0 0 0 0 0 0 0 0 0 0 0 0 0 0 0 |
Osteophorus roemeri |
1 0 1 0 0 0 0 0 0 1 1 0 0 1 1 1 0 1 1 0 3 1 1 1 0 1 0 ? ? ? ? ? ? ? ? ? ? ? ? ? ? ? ? ? ? ? ? ? ? ? ? ? ? ? ? ? ? 0 0 0 0 0 1 0 0 0 ? 0 1 0 ? |
Glaukerpeton avinoffi |
1 0 1 1 0 0 0 0 0 1 0 0 0 1 1 1 1 1 1 0 3 1 1 1 0 1 0 1 0 0 2 0 0 0 0 0 0 1 1 1 1 0 0 0 ? ? ? ? ? ? ? ? ? ? 0 0 0 0 0 0 0 0 1 0 0 0 1 0 0 0 0 |
Onchiodon langenhani |
1 0 1 1 0 0 0 0 0 1 0 0 0 1 1 1 1 1 1 0 3 1 1 1 0 1 0 1 0 0 2 0 0 0 0 0 0 1 1 1 1 0 0 0 1 0 0 1 0 0 1 0 2 2 0 1 0 0 0 0 0 0 1 0 0 0 ? 0 0 0 ? |
Onchiodon labyrinthicus |
1 0 1 1 0 0 0 0 0 1 0 0 0 1 1 1 1 0 1 0 3 1 1 1 0 1 0 1 0 0 2 0 0 0 0 0 0 1 1 1 1 0 0 0 0 0 0 1 0 0 1 0 2 2 0 0 0 0 0 0 0 0 0 0 1 0 1 0 ? 1 1 |
Onchiodon thuringiensis |
1 0 1 1 0 0 0 0 0 1 0 0 0 1 1 1 1 1 1 0 3 1 1 1 0 1 0 1 0 0 2 0 0 0 0 0 0 1 1 1 1 0 ? ? ? ? ? 1 0 0 1 0 2 2 0 1 0 0 0 0 0 0 1 0 1 0 1 0 0 1 1 |
Eryops megacephalus |
1 0 1 1 0 0 0 0 0 1 0 0 0 1 1 1 1 1 1 0 3 1 1 1 0 1 0 1 0 0 2 0 0 0 0 0 0 1 1 1 1 0 0 0 0 0 0 1 0 0 1 0 2 2 0 1 0 0 0 0 0 0 1 0 1 1 1 1 1 1 1 |
Eryops Moran |
1 0 1 1 0 0 0 0 0 0 0 0 0 1 1 1 1 1 1 0 3 1 1 1 0 1 0 1 0 0 2 0 0 0 0 0 0 1 1 1 1 0 0 0 0 0 0 1 0 0 1 0 2 2 0 1 0 0 0 0 0 0 1 0 1 1 1 1 0 1 1 |
Eryops anatinus |
1 0 1 1 0 0 0 0 0 1 0 0 0 1 1 1 1 1 1 0 3 1 1 1 0 1 0 1 ? ? ? ? ? ? ? ? ? ? ? ? ? ? ? ? ? ? ? ? ? ? ? ? ? ? ? 1 0 0 0 0 0 0 1 0 1 ? ? 1 1 1 ? |
Clamorosaurus nocturnus |
1 0 1 1 0 0 0 0 0 1 0 0 0 1 1 1 1 1 1 0 3 1 1 1 0 1 0 1 0 0 2 0 0 0 0 0 0 1 1 1 1 0 ? ? ? ? ? ? ? ? ? ? ? ? 0 1 0 0 0 0 0 0 1 0 1 1 1 0 1 1 1 |
“Sclerocephalus” stambergi |
1 0 1 1 0 0 0 0 0 0 1 1 1 0 0 1 0 0 0 ? 2 1 0 1 1 0 0 ? ? ? ? ? ? ? ? ? ? ? ? ? ? ? 1 1 1 ? ? ? ? ? ? ? ? ? ? ? 0 0 0 0 0 0 0 0 0 0 0 0 0 0 0 |
Sclerocephalus bavaricus |
1 1 0 1 0 0 0 0 0 0 1 1 2 0 0 1 0 0 0 1 2 1 0 1 1 1 0 1 0 0 0 0 ? 0 0 0 0 0 1 1 0 ? 1 0 1 0 0 1 ? 0 1 ? 1 2 ? ? 0 0 0 0 0 0 1 1 0 0 0 0 0 0 0 |
Sclerocephalus haeuseri |
1 0 1 1 0 0 0 0 0 1 1 2 2 0 0 1 0 0 0 0 2 1 0 1 1 1 0 1 0 0 0 0 1 0 0 0 0 0 1 1 0 1 1 0 1 0 0 1 0 0 1 0 1 2 1 0 1 0 0 0 0 0 1 1 0 0 0 0 0 0 0 |
Sclerocephalus concordiae |
1 0 1 1 0 0 0 0 0 1 1 2 2 0 0 1 0 0 0 0 2 1 0 1 1 1 0 1 0 0 0 0 1 0 0 0 0 1 1 1 0 1 1 0 1 0 0 1 0 0 1 ? 1 2 0 1 0 0 0 0 0 0 1 1 0 0 0 0 0 0 0 |
Glanochthon angusta |
1 1 1 1 1 0 0 0 1 1 1 1 ? 0 1 1 0 0 0 1 2 1 0 1 1 1 0 1 0 0 0 0 1 0 1 0 0 0 1 1 0 0 1 1 1 0 0 1 1 0 1 1 1 1 1 0 0 1 1 1 1 1 1 1 0 0 0 0 0 0 0 |
Glanochthon latirostre |
1 1 1 1 1 0 0 0 1 1 1 1 1 0 1 1 0 0 0 1 1 1 0 1 1 1 1 1 0 0 0 0 1 0 1 0 0 0 1 1 0 0 1 1 1 0 0 1 1 0 1 1 1 1 1 0 0 1 1 1 1 1 1 1 0 0 0 0 0 0 0 |
Intasuchus silvicola |
1 1 0 1 0 0 0 1 ? 0 0 0 0 0 1 1 0 1 0 ? 0 1 0 1 ? 1 1 1 0 0 0 1 1 1 1 0 1 0 1 1 0 ? ? ? ? ? ? ? ? ? ? ? ? ? 1 0 0 0 0 0 0 0 0 0 0 0 0 0 0 0 0 |
Melosaurus uralensis |
1 1 0 1 0 0 0 1 0 0 1 0 0 0 1 1 0 1 0 ? 1 1 0 1 ? 1 0 ? ? ? ? ? ? ? ? ? ? ? ? ? ? ? 1 1 ? 0 ? ? 1 ? ? ? ? ? 1 0 0 0 0 0 0 0 0 0 0 0 0 0 0 0 0 |
Cheliderpeton vranyi |
1 0 0 1 0 0 0 0 0 0 ? 0 0 0 0 1 0 1 0 1 2 1 0 1 ? 1 0 ? ? ? ? ? ? ? ? ? ? ? ? ? ? ? 1 0 1 0 0 1 1 0 1 1 2 1 1 0 0 0 0 0 0 0 0 0 0 0 0 0 0 0 0 |
Archegosaurus decheni |
1 1 0 1 0 1 0 1 0 0 1 0 0 0 1 1 0 1 0 1 2 1 0 1 1 1 1 1 1 0 0 1 1 1 1 1 1 0 1 1 0 1 1 1 1 0 0 1 1 0 1 1 1 1 1 0 0 0 0 1 0 0 0 0 0 0 0 0 0 0 0 |
Platyoposaurus stuckenbergi |
1 1 0 1 0 1 0 0 0 0 1 0 0 0 1 1 0 1 0 1 2 1 0 1 1 1 0 1 1 1 0 1 1 1 1 1 1 0 1 1 0 0 1 1 1 0 0 1 1 ? 1 1 1 1 1 0 0 0 0 0 0 0 0 0 0 0 0 0 0 0 0 |
Australerpeton cosgriffi |
1 1 0 1 0 1 0 0 0 0 1 0 0 0 1 1 0 1 0 1 2 1 0 1 1 1 0 1 1 1 0 1 1 1 1 1 1 1 1 1 0 0 1 1 1 0 0 1 0 ? 1 1 1 1 1 0 0 0 0 0 0 0 0 0 0 0 0 0 0 0 0 |
Table B1Data set (PCA).
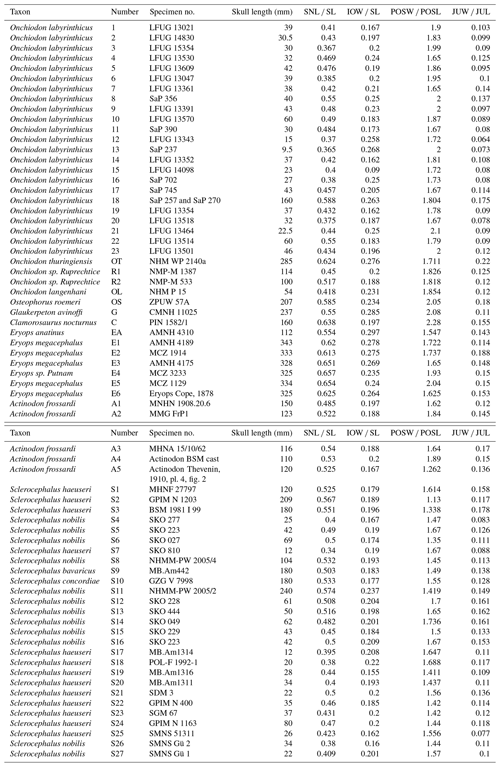
AMNH – American Museum of Natural History, New York; BSM – Bayerische Staatssammlung, Munich; CMNH – Carnegie Museum of Natural History, Pittsburgh; GPIM – Institute of Geosciences at the University of Mainz; GZG – Geowissenschaftliches Zentrum, Göttingen; LFUG – Landesamt für Umwelt, Landwirtschaft und Geologie, Freiberg; MCZ – Museum of Comparative Zoology, Harvard University, Cambridge, MA; MMG – Museum of Mineralogy and Geology, Dresden; MHNA – Muséum d'Histoire Naturelle, Autun; MNHN – Muséum National d'Histoire Naturelle, Paris; NHM – Naturhistorisches Museum, Schleusingen; NHMM – Naturhistorisches Museum Mainz; NMP – Narodní Muzeum, Prague; PIN – Paleontological Institute, Moscow; POL-F – Pollichia, Pfalzmuseum für Naturkunde, Bad Dürkheim; SDM – former Dreyer collection (now part of GPIM); SKO – Krätschmer collection, Odernheim; SMNS – Staatliches Museum für Naturkunde Stuttgart; ZPUW – Paleontological Institute at the University of Wroclaw.
The following free software packages were employed. TNT 1.5 is available under http://www.lillo.org.ar/phylogeny/tnt/ (Goloboff et al., 2003). PAST 4.01 (Hammer et al., 2001) is available under https://www.nhm.uio.no/english/research/infrastructure/past.
The complete data set is provided in Appendices A and B.
The author declares that there is no conflict of interest.
Publisher’s note: Copernicus Publications remains neutral with regard to jurisdictional claims in published maps and institutional affiliations.
I thank Isabell Rosin (Stuttgart) for skillfully producing casts; Axel Friebe and Jörg Schneider (Freiberg) as well as Ulf Linnemann (Dresden) for access to material; and Andrew Milner (London), Ralf Werneburg (Schleusingen) and Florian Witzmann (Berlin) for many helpful discussions. The three reviewers provided much helpful constructive criticism and many helpful suggestions.
This paper was edited by Torsten Scheyer and reviewed by Michael Buchwitz, Christian Klug and one anonymous referee.
Boy, J. A.: Die Branchiosaurier (Amphibia) des saarpfälzischen Rotliegenden (Perm, SW-Deutschland), Abh. Hess. L.-A. Bodenfor., 65, 1–137, 1972.
Boy, J. A.: Die Larven der rhachitomen Amphibien (Amphibia: Temnospondyli; Karbon-Trias), Paläontol. Z., 48, 236–268, 1974.
Boy, J. A.: Typen und Genese jungpaläozoischer Tetrapoden-Lagerstätten, Palaeontographica, 156, 111–167, 1977.
Boy, J. A.: Über einige Vertreter der Eryopoidea (Amphibia: Temnospondyli). 1. Sclerocephalus, Paläontol. Z., 62, 429–457, 1988.
Boy, J. A.: Uber einige Vertreter der Eryopoidea (Amphibia: Temnospondyli) aus dem europäischen Rotliegend (? höchstes Karbon–Perm). 2. Acanthostomatops, Paläontol. Z., 63, 133–151, 1989.
Boy, J. A.: Über einige Vertreter der Eryopoidea (Amphibia: Temnospondyli) aus dem europäischen Rotliegend (?höchstes Karbon-Perm). 3. Onchiodon, Paläontol. Z., 64, 287–312, 1990.
Boy, J. A.: Über einige Vertreter der Eryopoidea (Amphibia: Temnospondyli) aus dem europäischen Rotliegend (?höchstes Karbon-Perm). 4. Cheliderpeton latirostre, Paläontol. Z., 67, 123–143, 1993.
Boy, J. A.: Über die Micromelerpetontidae (Amphibia: Temnospondyli). 1. Morphologie und Paläoökologie des Micromelerpeton credneri (Unter-Perm; SW-Deutschland), Paläontol. Z., 69, 429–457, 1995.
Boy, J. A.: Paläoökologische Rekonstruktion von Wirbeltieren: Möglichkeiten und Grenzen, Paläontol. Z., 77, 123–152, 2003.
Broom, R.: Studies on the Permian temnospondylous Stegocephalia of North America, B. Am. Mus. Nat. Hist., 32, 563–595, 1913.
Bystrow, A. P. and Efremov, J. A.: Benthosuchus sushkini Efremov – a labyrinthodont from the Eotriassic of the Sharzenga River, Trudy Paleont. Inst., 10, 1–152, 1940.
Cope, E. D.: The rhachitomous Stegocephala, Am. Nat., 16, 334–335, 1882.
Credner, H.: Die Stegocephalen aus dem Rotliegenden des Plauen'schen Grundes bei Dresden, 3. Theil. Z. deut. geol. Ges., 34, 213–237, 1882.
Degani, G.: Cannibalism among Salamandra salamandra (L.) larvae, Israel J. Zool., 39, 125–129, 1993.
Duellman, W. and Trueb, L.: Biology of Amphibians, Johns Hopkins University Press, Baltimore, 1994.
Gaudry, A.: Memoire sur le reptile decouvert par M. Ch. Frossard à Muse (Saône-et-Loire), Nouv. Arch. Mus. Nation. d'Hist. Naturelle, Paris, 40 pp., 1867.
Gebhardt, U. and Schneider, J.: Palökologie und Paläobiogeographie “mariner” Kalkalgen im kontinental-lakustrischen Niederhäslich-Kalk des intermontanen Döhlen-Beckens(Unterrotliegend, Assel, Elbe-Zone), Freiberger Fh., C 450, 82–108, 1993.
Geinitz, H. B.: Dyas oder die Zechsteinformation und das Rotliegende, Teile 1–18, 342 pp., Selbstverlag, Dresden, 1862.
Goloboff, P., Farris, J., and Nixon, K.: TNT, “Tree analysis using New Technology”, Lillo [code], available at: http://www.lillo.org.ar/phylogeny/tnt/ (last access: 21 September 2021), 2003.
Gubin, Y. M.: The first eryopids from the Permian of the East European platform, Paleontol. J., 1983, 110–115, 1983.
Hammer, Ö., Harper, D. A. T., and Ryan, P. D.: PAST: Paleontological statistics software package for education and data analysis, Palaeontol. Electron., 4, 1–9, 2001 (code available at: https://www.nhm.uio.no/english/research/infrastructure/past, last access: 21 September 2021).
Hoffman, E. A. and Pfennig, D. W.: Proximate causes of cannibalistic polyphenism in larval tiger salamanders, Evolution, 80, 1076–1080, 1999.
Klembara, J. and Steyer, J. S.: A new species of Sclerocephalus (Temnospondyli: Stereospondylomorpha) from the early Permian of the Boskovice Basin (Czech Republic), J. Paleontol., 86, 302–310, 2012.
Marsh, O. C.: Notice of new fossil reptiles, Am. J. Sci., 15, 409–411, 1878.
Meckert, D.: Der Schultergürtel des Sclerocephalus haeuseri Goldfuss, 1849 im Vergleich mit Eryops Cope, 1877 (Eryopoidea, Amphibia, Perm), Palaeontogr. Abt. A, 229, 113–140, 1993.
Meyer, H. v.: Osteophorus roemeri aus dem Rothliegenden von Klein-Neundorf in Schlesien, Palaeontographica, 7, 99–104, 1860.
Michimae, H.: Differentiated phenotypic plasticity in larvae of the cannibalistic salamander Hynobius retardatus, Behav. Ecol. Sociobiol., 60, 205–211, 2006.
Miner, R. W.: The pectoral limb of Eryops and other primitive tetrapods, B. Am. Mus. Nat. Hist., 41, 145–312, 1925.
Pawley, K. and Warren, A. A.: The appendicular skeleton of Eryops megacephalus Cope, 1877 (Temnospondyli: Eryopoidea) from the Lower Permian of North America, J. Paleontol., 80, 561–580, 2006.
Reichel, W.: Fossilführung, Taphozönosen und Biostratigraphie des Döhlener Beckens, in: Das Döhlener Becken bei Dresden. Geologie und Bergbau, Bergbau in Sachsen, edited by: Reichel, W. and Schauer, M., 12, 16–42, Sächsisches Landesamt für Umwelt und Geologie (LfUG), Freiberg, 2006.
Reilly, S. M., Wiley, E. O., and Meinhardt, D. J.: An integrative approach to heterochrony: the distinction between interspecific and intraspecific phenomena, Biol. J. Linn. Soc., 60, 119–143, 1997.
Romer, A. S.: Vertebrate faunal horizons in the Texas Permo-Carboniferous red beds, Univ. Texas Bull., 2801, 67–108, 1928.
Romer, A. S.: Early history of the Texas redbed vertebrates, Geol. Soc. Bull., 46, 1597–1658, 1935.
Romer, A. S.: Notes on branchiosaurs, Am. J. Sci., 237, 748–761, 1939.
Romer, A. S.: Late Pennsylvanian and early Permian vertebrates in the Pittsburgh-West Virginia region, Ann. Carnegie Mus., 33, 47–113, 1952.
Sander, P. M.: Early Permian depositional environments and pond bonebeds in Central Archer County, Texas, Palaeogeogr. Palaeocl., 69, 1–21, 1989.
Sawin, H. J.: The cranial anatomy of Eryops megacephalus, Bull. Mus. Comp. Zool., Harvard Coll., 88, 405–464, 1941.
Schlichting, C. D. and Pigliucci, M.: Phenotypic Evolution. A reaction norm perspective, Sinauer, Sunderland, 1998.
Schneider, J.: Environment, biotas and taphonomy of the Lower Permian lacustrine Niederhäslich limestone, Döhlen basin, Germany, Trans. Royal Soc. Edinburgh Earth Sci., 84, 453–464, 1993.
Schoch, R. R.: The evolution of life cycles in extinct amphibians, Annu. Rev. Earth Planet. Sc., 37, 135–162, 2009.
Schoch, R. R.: The major clades of temnospondyls: an inclusive phylogenetic analysis, J. Syst. Palaeontol., 11, 673–705, 2013.
Schoch, R. R.: Life cycles, plasticity, and palaeoecology in temnospondyl amphibians, Palaeontology, 57, 517–529, 2014a.
Schoch, R. R.: Amphibian Evolution. The Life of Early Land Vertebrates, Wiley, New Jersey, 288 pp., 2014b.
Schoch, R. R. and Fröbisch, N. B.: Metamorphosis and neoteny: alternative developmental pathways in an extinct amhibian clade, Evolution, 60, 1467–1475, 2006.
Schoch, R. R. and Milner, A. R.: Temnospondyli, in: Encyclopedia of Paleoherpetology, edited by: Sues, H. D., 3A2. Pfeil, Munich, 2014.
Schoch, R. R. and Sobral, G.: A new species of Sclerocephalus with a fully ossified endocranium gives insight into braincase evolution in temnospondyls, J. Paleontol., 2021.
Schoch, R. R. and Witzmann, F.: Osteology and relationships of the temnospondyl genus Sclerocephalus, Zool. J. Linn. Soc., 157, 135–168, 2009a.
Schoch, R. R. and Witzmann, F.: The temnospondyl genus Glanochthon from the Meisenheim Formation, Spec. Pap. Palaeont., 81, 121–136, 2009b.
Schoch, R. R., Fastnacht, M., Fichter, J., and Keller, T.: Anatomy and relationships of the Triassic temnospondyl Sclerothorax, Acta Palaeontol. Polon., 52, 117–136, 2007.
Steyer, J. S.: Ontogeny and phylogeny in temnospondyls: a new method of analysis, Zool. J. Linn. Soc., 130, 449-467, 2000.
Thevenin, A.: Les plus anciens quadrupèdes de France, Ann. Paléontol., 5, 1–63, 1910.
Watson, D. M. S.: The structure, evolution, and origin of the Amphibia. The orders Rachitomi [sic] and Stereospondyli, Philos. T. Roy. Soc. Lond. B, 209, 1–73, 1919.
Werneburg, R.: Die Amphibienfauna der Manebacher Schichten (Unterrotliegendes, Unterperm) des Thüringer Waldes, Veröff. Naturhist. Mus. Schleusingen, 4, 55–68, 1989.
Werneburg, R.: Die Branchiosaurier aus dem Unterrotliegend des Döhlener Beckens bei Dresden, Veröff. Naturhist. Mus. Schleusingen, 6, 75–99, 1991.
Werneburg, R.: Onchiodon (Eryopidae, Amphibia) aus dem Rotliegend des Innersudetischen Beckens (Böhmen), Paläontol. Z., 67, 343–355, 1993.
Werneburg, R.: Temnospondyle Amphibien aus dem Karbon Mitteldeutschlands, Veröff. Naturhist. Mus. Schleusingen, 11, 23–64, 1996.
Werneburg, R.: Der Eryopide Onchiodon (Amphibia) aus dem Rotliegend des Beckens von Autun (Frankreich), Freiberger Fh., C 466, 167–181, 1997.
Werneburg, R.: Der “Manebacher Saurier” – ein neuer großer Eryopide (Onchiodon) aus dem Rotliegend (Unter-Perm) des Thüringer Waldes, Veröff. Naturhist. Mus. Schleusingen, 22, 3–40, 2008.
Werneburg, R. and Berman, D. S.: Revision of the aquatic eryopid temnospondyl Glaukerpeton avinoffi Romer, 1952, from the Upper Pennsylvanian of North America, Ann. Carnegie Mus., 81, 33–60, 2012.
Werneburg, R. and Steyer, J. S.: Redescription of the holotype of Actinodon frossardi (Amphibia, Temnospondyli) from the Lower Permian of the Autun basin (France), Geobios, 32, 599–607, 1999.
Werneburg, R., Stamberg, S., and Steyer, J. S.: A new stereospondylomorph, Korkonerpeton kalnense gen. et sp. Nov. From the Lower Permian of the Czech Krkonose Piedmont Basin, and a redescription of Intasuchus silvicola from the Lower Permian of Russia (Temnospondyli, Amphibia), Fossil Imprint, 76, 217–242, 2020.
Wiens, J. F., Bonett, R. M., and Chippindale, P. T.: Ontogeny discombobulates phylogeny: paedomorphosis and higher-level salamander relationships, Syst. Biol., 54, 91–110, 2005.
Witzmann, F.: The external gills of Palaeozoic amphibians, N. Jb. Geol. Paläont. Abh., 232, 375–401, 2004.
Witzmann, F.: Hyobranchial and postcranial ontogeny of the temnospondyl Onchiodon labyrinthicus (Geinitz, 1861) from Niederhäslich (Döhlen Basin, Autunian, Saxony), Paläontol. Z., 79, 479–492, 2005.
Witzmann, F.: Morphology and palaeobiology of the Permo-Carboniferous temnospondyl amphibian Archegosaurus decheni Goldfuss, 1847 from the Saar-Nahe Basin, Germany, Trans. Royal Soc. Edinburgh Earth Sci., 96, 131–162, 2006a.
Witzmann, F.: Developmental patterns and ossification sequence in the Permo-Carboniferous temnospondyl Archegosaurus decheni (Saar-Nahe Basin, Germany), J. Vert. Paleontol., 26, 7–17, 2006b.
Witzmann, F.: Cannibalism in a small growth stage of the Early Permian branchiosaurid Apateon gracilis (Credner, 1881) from Saxony, Foss. Rec., 12, 7–11, https://doi.org/10.1002/mmng.200800006, 2009.
Witzmann, F. and Schoch, R. R.: Skeletal development of the temnospondyl Acanthostomatops vorax from the Lower Permian Döhlen Basin of Saxony, Trans. Royal Soc. Edinburgh Earth Sci., 96, 365–185, 2006.
Witzmann, F. and Pfretzschner, H. U.: Larval ontogeny of Micromelerpeton credneri (Temnospondyli, Dissorophoidea), J. Vertebr. Paleontol., 23, 750–768, 2003.
Zittel, K. A. v.: Handbuch der Palaeontologie: I. Abtheilung. Palaeozoologie. III. Band. Vertebrata (Pisces, Amphibia, Reptilia, Aves), 900 pp., Berlin, 1888.